LASER COOLING: Optical refrigerator cools semiconductor payload to 165 K
In optical refrigeration, laser light creates anti-Stokes fluorescence upconversion in a material, which carries heat away from the material. Earlier this year, a group at the University of New Mexico and the Universita di Pisà (Pisa, Italy) demonstrated laser cooling of a ytterbium-doped yttrium lithium fluoride (Yb:YLF) crystal to 155 K; however, only the crystal was cooled, and not a useful "payload" in addition.1
Now, the same researchers have used a Yb:YLF crystal to cool a useful semiconductor payload to 165 K.2 This is important because at present, relatively easy-to-use thermoelectric coolers can only cool down to 170 K and thus reaching lower temperatures requires the use of much more complex cryogenic cooling.
A realistic payload
The payload was a 2 μm thick gallium arsenide semiconductor passivated by a gallium indium phosphide coating, with a double-heterostructure geometry having a high external quantum efficiency (in other words, it was similar to real-life semiconductor devices, some of which need cooling). It weighed 5 μg, was adhesive-contacted to the Yb:YLF crystal, and was optically transparent at the 1023 nm laser wavelength used for the experiment.
The payload and cooling crystal were placed in a vacuum chamber; a 9 W continuous-wave Yb:YAG thin-disk laser supplied light to the cooling crystal in a nonresonant cavity geometry. A small amount of light from an outside laser diode was coupled into the semiconductor structure to measure its temperature via the shift in bandgap. The temperature of the Yb:YLF crystal itself was measured by monitoring its fluorescence spectrum.
As would be expected, the cooling power of the setup is greatest when the crystal is at room temperature, and declines as its temperature drops. Conversely, at room temperature the cooling load (the amount of heat that must be removed from the crystal to keep it at its current temperature) is zero and rises as the temperature cools. At the temperature where the load and the power are the same, the crystal and its payload can be cooled no further (see figure). At room temperature (300 K), the cooling power of the setup was 140 mW, which dropped to 20 mW at 165 K.
The researchers believe that by reducing radiative load and improving crystal material quality, temperatures down near that of liquid nitrogen (77 K) could be reached.
REFERENCES
1. D.V. Seletskiy et al., Nature Photon., 4, 3, 161 (2010).
2. D.V. Seletskiy et al., Opt. Exp., 18, 17, 18061 (Aug. 16, 2010).
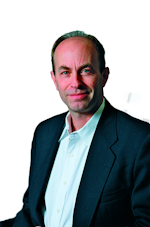
John Wallace | Senior Technical Editor (1998-2022)
John Wallace was with Laser Focus World for nearly 25 years, retiring in late June 2022. He obtained a bachelor's degree in mechanical engineering and physics at Rutgers University and a master's in optical engineering at the University of Rochester. Before becoming an editor, John worked as an engineer at RCA, Exxon, Eastman Kodak, and GCA Corporation.