PHOTONIC FRONTIERS: EYE-SAFE LASERS - Retina-safe wavelengths benefit open-air applications
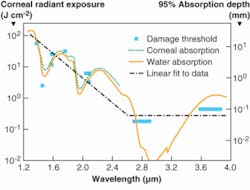
Eye safety can be a tough problem when laser beams must be transmitted through the open air. It’s difficult to completely avoid human exposure in applications such as laser radar, remote sensing, rangefinding, target designation, laser countermeasures, and high-energy laser weapons. So the use of lasers at so-called “eye-safe wavelengths,” particularly 1.4 to 1.8 µm, is increasing. At these wavelengths, maximum permissible eye exposures are much higher than in the visible or on the 1.06 µm neodymium line.
Military agencies have been at the forefront of studying eye hazards and developing lasers that emit at wavelengths that reduce eye hazards to their own troops and bystanders during training exercises, war games, and even combat. Civilian systems that send beams through open air, such as golf rangefinders, can function at low powers, but military systems need more power, so they can benefit most from shifting to wavelengths where maximum permissible exposures are higher.
What are ‘eye-safe’ wavelengths?
Safety regulations define maximum permissible exposures based on how laser light affects the eye. Water absorption in eye tissue and the intraocular fluid blocks light at wavelengths longer than 1.4 µm from reaching the retina, the part of the eye most vulnerable to laser damage. However, safety specialists warn that lasers are not “eye safe” merely because they emit beyond 1.4 µm. High powers at those wavelengths can damage the cornea, particularly at water absorption peaks near 3 and 10 µm. To Wesley Marshall, manager of the laser/optical radiation program at the Army Center for Health Promotion and Preventative Medicine (Aberdeen Proving Ground, MD), the only “eye-safe lasers” are those that meet the stringent emission requirements for Class 1.
Bruce Stuck of the Army Medical Research Detachment (Brooks AFB, TX) prefers the term “retina safe” for lasers emitting beyond 1.4 µm, reflecting a dividing point in current safety standards. Maximum permissible exposures for wavelengths shorter than 1.4 µm, where the light can reach the retina, are low but allowable exposures jump at 1.4 µm to the much higher levels needed to cause corneal damage.
Those standards are likely to be refined after recent studies revealed that complex eye hazards in the 1.4 µm region don’t change as abruptly with wavelength as existing standards assume. The cornea and lens are vulnerable to internal heating by absorbed laser energy that varies with material absorption, pulse duration, and repetition rate as well as power. Experiments show that the threshold for corneal damage decreases with the absorption depth of corneal tissue at that wavelength (see Fig. 1). Measured corneal absorption is close to that of water, which makes up about 70% of corneal tissue, and increases steadily from 1.2 to 2.9 µm.
Strong absorption concentrates heating in a thin surface zone with consequent risk of damage (see Fig. 2). Strong absorption at the 10.6 µm wavelength of carbon dioxide lasers deposits potentially dangerous energy in the epithelium, the outer layer of the cornea. Absorption is much lower at 1.54 µm, so light penetrates more deeply, distributing the heat through a larger volume, and making corneal damage much less likely than at 10.6 µm. However, Russ McCally of the Johns Hopkins University Applied Physics Laboratory (Laurel, MD) recently found that at 1.54 µm the damage threshold for the endothelium, the layer at the back of the cornea, is only slightly below that for the corneal surface damage.1 He says that’s worrisome because endothelial damage does not heal in humans, unlike corneal surface damage.The cornea can tolerate exposure to single pulses near 1.5 µm because heating is distributed, but at high repetition rates the heat can reach damaging levels, says Marshall. The wavelength where the risk of corneal damage exceeds retinal risk depends on exposure duration, increasing from 1.22 µm at long exposures to 1.45 µm for short exposes, says Stuck. His group has proposed revising standards to account for these effects, and remove the step-function jumps in permissible exposure standards.2
Recent studies of the 1.315 µm wavelength of the chemical oxygen-iodine laser (COIL) used in the Airborne Laser and the Advanced Tactical Laser (see www.laserfocusworld.com/articles/318547) show particularly complex effects. “You can create damage to the eye at any location between the cornea and the retina” by modest changes in experimental parameters, says Marshall. That makes it hard to establish new maximum permissible exposures for new laser weapons.
Options for retina-safe lasers
Lasers at retina-safe wavelengths have replaced neodymium lasers for stand-alone rangefinders in developed countries. Change is slower for rangefinders incorporated into other systems, and target designators are hard to shift to safer wavelengths when arsenals are filled with weapons built to hit targets marked at 1.06 µm.
Erbium-fiber and diode lasers are compact and efficient emitters at 1.54 µm, but their pulse energy is limited. They can measure intermediate distances by averaging returns over 1000 pulses, but 10 mJ pulses are needed for long ranges, especially in hostile environments. Today that’s the realm of erbium-glass lasers or optical parametric oscillators pumped with neodymium lasers. Raman shifting lags behind because reaching the 1.5 µm band requires either cumbersome high-pressure Raman cells pumped by neodymium lasers, or novel pump lasers for solid-state Raman modules.
First demonstrated in 1965 by Elias Snitzer, the erbium-glass laser typically is sensitized by ytterbium, which absorbs pump light and transfers the energy to erbium atoms.3 Erbium-glass emits directly at 1.54 µm and can produce energetic pulses. However, glass dissipates heat slowly, seriously restricting repetition rate.
For higher repetition rates and powers, a flashlamp-pumped Nd:YAG laser can pump an optical parametric oscillator (OPO) emitting at 1.57 µm. Northrop Grumman Laser Systems (Apopka, FL) produces a flashlamp-pumped KTP (potassium titanyl phosphate) optical module as small as a roll of quarters, says Jeff Cavins, advanced programs manager. The company is working on diode pumping the Nd:YAG laser to increase energy efficiency, an important concern for military field equipment.
Others have already demonstrated diode pumping, but pulse energy was limited to tens of microjoules. Last year Jieguang Miao of the Changchun Institute of Optics, Fine Mechanics, and Physics (Changchun, China) reported pumping a Q-switched Nd:YVO4 (vanadate) laser with 4 W of diode output, and using it to pump an OPO emitting average power of 580 mW at 1.57 µm. But with a repetition rate of tens of kilohertz, the pulse energy was limited.4 Erbium:YAG resonantly pumped with a diode or fiber laser to excite erbium atoms directly to the upper laser level can emit at 1.617 or 1.645 µm when the excited atoms drop to lower levels just above the ground state. Quantum efficiency can be quite high, and BAE Systems (Nashua, NH) has produced Q-switched pulses of more than 40 mJ.5 The technology is not as mature as Nd:YAG-pumped OPOs, but is attractively simple, says Scott Setzler of BAE. The Er:YAG lasers are already being used in developmental laser radars.
Eye safety is particularly critical for defense systems likely to be used in civilian areas, such as laser countermeasures to protect aircraft against shoulder-launched heat-seeking missiles. These systems operate in the 3 to 5 µm mid-infrared band, where permissible exposure limits are lower than at 1.5 µm, but well above the limits at 1.06 µm. Holmium lasers pumping zinc germanium phosphide OPOs “have opened up the 3 to 5 µm region,” says Evan Chicklis of BAE.
Applications and outlook
Retina-safe wavelengths allow higher exposures, but corneal burns remain a concern. Although most corneal injuries will heal, McCally warns that they are “exceedingly painful and disabling.” He also cites important gaps in our understanding, particularly the lack of data on picosecond and femtosecond pulses. Ultrashort pulses can cause nonlinear effects, he says, so “it wouldn’t be justified to extrapolate from standards developed in the nanosecond range.”
Lasers at retina-safe wavelengths can’t yet match the cost and performance of tried and tested neodymium lasers, but they are adequate for most applications and offer important benefits in easing eye-exposure limits. Relaxing exposure limits is a big plus for laser radars and other applications where beams may enter civilian environmentsparticularly in adding laser countermeasures to civilian aircraft. However, replacing existing target designators would raise logistical nightmares of arsenals with two incompatible generations of laser target designators and laser-guided bombs.
The toughest eye-safety issues will come as the Pentagon grapples with developing laser weapons. One clear advantage the chemical oxygen-iodine laser has over solid-state neodymium or ytterbium-fiber lasers is that maximum permissible exposure limits at 1.315 µm are an order of magnitude higher than at 1.06 or 1.1 µm. It’s interesting to wonder if the eye-safety advantage of the longer gas-laser wavelength would offset the logistic advantages of solid-state lasers emitting at shorter and more hazardous wavelengths.
REFERENCES
1. R. McCally et al., Health Physics 92, 205 (March 2007).
2. J. Zuclich, D. Lund, and B. Stuck, “Wavelength dependence of ocular damage thresholds in the near-IR to far-IR transition region: proposed revisions to MPEs” Health Physics 92, 15 (January 2007).
3. E. Snitzer and R. Woodcock, Appl. Phys. Lett. 6(3) 45 (February 1965).
4. J. Miao et al., Appl. Physics B: Lasers and Physics 88, 193 (2007).
5. S. Setzler et al., IEEE J. Selected Topics Quant. Electronics 11, 645 (May-Jun 2006).
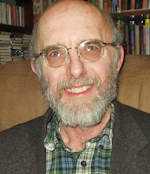
Jeff Hecht | Contributing Editor
Jeff Hecht is a regular contributing editor to Laser Focus World and has been covering the laser industry for 35 years. A prolific book author, Jeff's published works include “Understanding Fiber Optics,” “Understanding Lasers,” “The Laser Guidebook,” and “Beam Weapons: The Next Arms Race.” He also has written books on the histories of lasers and fiber optics, including “City of Light: The Story of Fiber Optics,” and “Beam: The Race to Make the Laser.” Find out more at jeffhecht.com.