LASER COOLING: Lasers make quantum wells ‘cool’
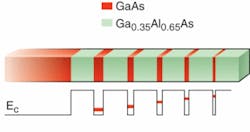
Lasers are widely known for their use in industrial applications for cutting through metals, or for their potential as defensive weapons: they bring to mind powerful, visible, searing beams. It’s atypical for lasers to connote cooling. But a team of researchers at Technische Universität Dortmund (Dortmund, Germany) and Ruhr-Universität Bochum (Bochum, Germany) have made initial steps toward changing this perception and using laser light to cool a semiconductor material.1 In this process, known as photoluminescence upconversion, the wavelength of the laser beam is carefully chosen so that the irradiated material relinquishes some of its energy to the beam. The photons in the laser beam absorb some of the oscillation energy of the particles (phonons) in the material, reducing the temperature of the target (see www.laserfocusworld.com/articles/28438). While this cooling effect had been examined extensively in its application to atomic vapor and condensed matter, such as dye solutions and glass, its effectiveness with another type of condensed matter—semiconductor materials—had not been established.
In a detailed experimental study, Soheyla Eshlaghi and colleagues examined how the laser-induced photoluminescence cooling of semiconductor quantum wells (QWs) changed over a wide range of sample temperatures from that of liquid helium up to room temperature. The samples in the study featured undoped gallium arsenide (GaAs) QWs varying in thickness from 2.8 to 39.3 nm, separated by 31-nm-thick aluminum gallium arsenide (Ga0.35Al0.65As) barriers. The group used an Oxford ITC 502 temperature controller and heater to vary the temperature of the samples from 4 K up to room temperature, and a GaAlAs sensor mounted near the sample to monitor the temperature. They directed a CW Ti:sapphire laser with a tunable wavelength of around 800 nm onto the surface of the sample with an intensity of about 50 W/cm2, which induced varying levels of anti-Stokes PL in the GaAs quantum wells.
The group focused on the 11.7-nm-thick quantum well, which featured the most dominant excitonic effects in the PL spectra at a temperature T of less than 80 K and thermal energy kBT of less than 7 meV (kB is Boltzmann’s constant). For measurements above 20 K in temperature, the highest cooling rate was obtained for laser detunings approximating an energy of ∆E = 2 kBT, in which detuning means shifting of the laser wavelength to a photon energy below the resonant energy of the quantum well.
The experiment had several other significant results. The team discovered that the anti-Stokes photoluminescence induced to cool the structure is temperature dependentin other words, the cooling efficiency of lasers in general increases with the temperature of the sample, like that of other cooling systems. Furthermore, the results enable calculation of the ideal laser wavelength as a function of temperature. Ultimately, controlling the sample temperature in concert with a carefully chosen laser wavelength can optimize the laser-cooling efficiency.
The precision and low cost of semiconductor production is a significant advantage to the technique of using lasers to cool semiconductors. Not only may vibration-free cooling of semiconductors become practical but the laser could someday be used to cool other lasers.
REFERENCE
1. S. Eshlaghi et al., Physical Review B 77, 245317 (2008).
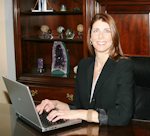
Valerie Coffey-Rosich | Contributing Editor
Valerie Coffey-Rosich is a freelance science and technology writer and editor and a contributing editor for Laser Focus World; she previously served as an Associate Technical Editor (2000-2003) and a Senior Technical Editor (2007-2008) for Laser Focus World.
Valerie holds a BS in physics from the University of Nevada, Reno, and an MA in astronomy from Boston University. She specializes in editing and writing about optics, photonics, astronomy, and physics in academic, reference, and business-to-business publications. In addition to Laser Focus World, her work has appeared online and in print for clients such as the American Institute of Physics, American Heritage Dictionary, BioPhotonics, Encyclopedia Britannica, EuroPhotonics, the Optical Society of America, Photonics Focus, Photonics Spectra, Sky & Telescope, and many others. She is based in Palm Springs, California.