Cell Biology/Optogenetics: Optogenetics method leverages CRISPR/Cas9 tools
Understanding gene function during cell growth, differentiation, metabolism, and homeostasis calls for tools able to control target gene expression in real time. Researchers at Texas A&M University have reported new progress in this area—progress that is promising for regenerative medicine, among other applications.
Over the past few years, the nuclease known as clustered regularly interspaced short palindromic repeats (CRISPR)-associated-9 (Cas9) has become recognized as a powerful genome-editing tool. Even more recently, researchers have engineered a catalytically inactive Cas9, known as dCas9, to target endogenous gene regulation without genetically altering DNA sequences. But without the ability to provide temporal control, these tools can cause off-target effects and restrict reversibility.
The researchers have developed synthetic chemical and light-sensitive dCas9 nuclear translocation systems to overcome this limitation, and to harness for optogenetic control the many calcium signals that regulate cell processes.
Their design pairs calcium ions (Ca2+)—both genetically encoded photoactivatable Ca2+ actuators and an engineered Ca2+-responsive transcriptional factor—with dCas9-effector fusions. The most effective of the solutions they’ve investigated uses a photoswitchable Ca2+ actuator engineered from calcium release-activated channels (CRAC). The approach allows remote control over calcium signals and Ca2+- dependent nuclear translocation of engineered dCas9 fusions.
Filling in with CRAC
The work builds upon the researchers’ earlier efforts to overcome limitations of prior channelrhodopsin-based optogenetic tools. These tools lack strict ion selectivity, as well as sensitivity in the near-infrared (near-IR) transmissible range that enables deeper tissue penetration. Their Opto-CRAC optogenetic platform leverages near-IR to selectively and remotely control Ca2+ oscillations and Ca2+-responsive gene expression.1 Thus, it enables regulation of nonexcitable cells, including T lymphocytes, macrophages, and dendritic cells.
The researchers use upconverting nanoparticles to enable such in vivo applications as remote-controlled innate and adaptive immune responses. The nanoparticles’ ability to shift the optogenetic operation window from visible to near-IR wavelengths enables wireless photoactivation of Ca2+-dependent signaling and optogenetic modulation of immunoinflammatory responses.
Reprogramming transcription with light
The team’s latest invention, called CaRROT (calcium-responsive transcriptional reprogramming tool), is designed to hijack light-generated calcium signals to deliver the genome-engineering tool derived from CRISPR/Cas9 (see figure).2 Yubin Zhou, associate professor at Texas A&M’s Institute of Biosciences and Technology, led development of CaRROT, which enables high-precision control of gene transcription, dictating how, when, and where genes create proteins that perform various functions.
CaRROT uses pulsed light or chemicals to induce the flow of calcium ions into cells. When the light is switched on, the gates controlling calcium ions open to allow calcium into the cell’s cytoplasm, says Nhung Nguyen, a graduate student who led this work. This process ultimately triggers the expression of specific genes, which leads to functional changes in the cell.
The team screened dozens of engineered proteins and optimized CaRROT for responsiveness to light, adds Lian He, Ph.D., a graduate student in Zhou’s lab and a co-first author of the study. To evaluate CaRROT’s effectiveness in mammalian cells, the team will test it on genes that control the differentiation of neuron and skeletal muscle.
Ultimately, they hope to use CaRROT to drive the precise differentiation of stem cells for regenerative medicine and thus to grow any organ needed by illuminating cells with light. “The improvement of light penetration in deep tissue gives us the optimism that we could use CaRROT to reprogram cells in damaged organs,” says Yun Huang, Ph.D., a collaborative senior author of the study. “It is possible that one day, by just exposing the tissues to light, we can heal the wound or accelerate the regeneration of injured tissues by phototuning coordinated gene expression.”
Turning off for treatment
The researchers have also devised an optogenetic tool to turn off calcium influx through cell-membrane gateways called voltage-gated calcium channels.3 Because dysfunction of these channels in the cells of “excitable” tissues such as the nervous and cardiovascular systems is involved in many diseases, they are considered an important therapeutic target.
Traditional calcium-channel blockers approved by the U.S. FDA are widely used to treat such cardiovascular disorders as high blood pressure, arrhythmia, and coronary artery disease. But patients using these drugs commonly report headaches, edema, dangerously low blood pressure, and palpitations, thanks to cytotoxicity and off-target effects. “Our new optogenetic tool provides a non-conventional method to interrogate physiological and pathophysiological processes medicated by these voltage-gated calcium channels,” Zhou says.
His team engineered a class of genetically encoded inhibitors for these voltage-gated calcium channels, the best of which, optoRGK, shows excellent light-inducible inhibition of Ca2+ entry in excitable cells, according to assistant project scientist Guolin Ma, who spearheaded this work. The researchers tested this tool in cardiac muscle cells, which showed a substantial reduction or termination of rhythmic calcium oscillation in response to blue light illumination and then reversal upon removal of the light.
While the traditional voltage-gated calcium channel blockers lack reversibility, selectivity, and tissue specificity, optoRGK promises unprecedented precision, and together with the researchers’ other tools, sets the stage for convenient control of numerous processes in multiple biological systems. The team hopes that their studies will eventually lead to a new generation of optogenetic devices for curing cancer, cardiovascular, and neurological diseases.
REFERENCES
1. L. He et al., eLife, 4, e10024 (2015); doi:10.7554/elife.10024.
2. N. T. Nguyen et al., ACS Synth. Biol., 7, 814−821 (2018).
3. G. Ma et al., Angew. Chem. Int. Ed. Engl., doi:10.1002/anie.201713080 (2018).
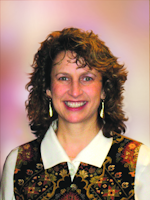
Barbara Gefvert | Editor-in-Chief, BioOptics World (2008-2020)
Barbara G. Gefvert has been a science and technology editor and writer since 1987, and served as editor in chief on multiple publications, including Sensors magazine for nearly a decade.