Translational Research/Microendoscopy: Multimodal endoscopy targets real-time biopsy
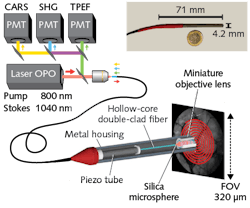
Tissue biopsy is a key early step in the diagnosis and treatment of many types of cancer. Under the current standard, tissue samples are surgically retrieved and sent to a pathology laboratory for microscopic analysis, which generates results within a few days. Clearly, it would be highly advantageous to analyze tissue in situ—for instance, during endoscopic probing. But obtaining sectional images with the requisite contrast and resolution—using a remote probe and without the staining labels—presents numerous challenges.
Recently, however, research at the Institut Fresnel (Marseille, France) has demonstrated the ability to perform multimodal imaging at high resolution using a unique endoscopic probe based on a double-clad hollow-core fiber together with a turnkey femtosecond laser. The work potentially paves the way to intraoperative, label-free imaging for real-time histopathology diagnosis and surgical guidance.
Biopsy information
Histopathology is the process of analyzing tissue samples under a microscope to identify abnormalities indicating disease. Histopathology imaging processes are well established—the retrieved tissue sample is chemically fixed with formalin to stabilize its structure, and then successively dehydrated and embedded in paraffin wax. (Alternatively, freezing is commonly used in place of fixing and wax processing.) The sample is then carefully sectioned into thin slices, which are then stained by a group of dyes or labels (with a growing number of customized antibodies increasingly also used) that preferentially bind to cell nuclei, cytoplasm, collagen, or antigens.
With this combination, the histopathologist can distinguish details of cell bodies and their nuclei, as well as structures of the extra-cellular matrix—details that enable recognition of abnormalities present with cancer and other serious diseases.
The major disadvantage of this approach is that at least a few days pass between surgical sample retrieval and diagnosis, which delays treatment in the case that test results are positive. Furthermore, this traditional approach subjects the patient to multiple procedures if surgical removal of the tumor or tissue is called for. This causes additional delay, increases treatment cost, and can be problematic for patients who are poor surgical candidates.
Multimodal nonlinear imaging
These limitations would be eliminated if intraoperative imaging could provide the same information delivered by histopathology. Several capabilities are required of an imaging method able to achieve this goal: the method must be able to perform optical sectioning (which mimics the physical microtome slicing done in the lab) and differentiate various tissue components to highlight their morphologies, without dyes and fluorescent labels. It must also be compatible with the manual port of an endoscope, and able to provide real-time imaging performance.
The need to perform optical sectioning (that is, three-dimensional imaging) in real time dictates the use of a confocal or nonlinear microscopy method. While confocal devices with endoscopic compatibility already exist, confocal imaging generally requires the introduction of fluorescent dyes and labels to obtain all the information needed for histopathological screening. This leaves nonlinear microscopy with ultrafast laser pulses as the only appropriate tool for high-resolution, label-free imaging.
Of the many nonlinear microscopy methods available, a few—those based on two-photon auto-fluorescence (TPF) excitation, second harmonic generation (SHG), and stimulated Raman—are known to provide the most useful label-free images. Such approaches also provide specificity. Coherent anti-Stokes Raman scattering (CARS) and stimulated Raman scattering (SRS) can image lipids and proteins. And with sufficient spectral resolution, Raman imaging could in principle be tuned to distinguish lipids (via =CH2 vibrations) from proteins (via –CH3 vibrations). Meanwhile, SHG is well proven for imaging collagen and, hence, the extracellular matrix.
Thus, the combination of two coherent Raman wavelengths and an SHG image could provide images in which the cell bodies, their nuclei, and the extracellular matrix are all clearly visible at the requisite resolution. Moreover, a combination of two laser wavelengths could enable generation of all three images.
Short pulses, hollow core
Researchers at Institut Fresnel have demonstrated a practical and viable endoscopic tool with just these capabilities.
Turnkey laser sources easily provide the separate pump and Stokes pulses wavelengths required for coherent Raman over a wide spectral range. The researchers’ demonstration uses a tunable, multiwavelength laser system (Coherent Discovery) based on an ytterbium (Yb) fiber laser amplifier and an integrated tunable optical parametric oscillator. This integrated, one-box system provides two synchronized ultrashort 80 MHz pulse trains: 100 fs pulses tuned to 800 nm to serve as the CARS pump wavelength, and 140 fs pulses at 1040 nm for the Stokes. The difference in these wavelengths is considered ideal for CARS imaging of carbon hydrogen (CH2 and CH3) bonds (~2885 cm-1).
For endoscopic compatibility, transmission of these femtosecond pulses requires single-mode fiber to preserve pulse shape and enable the small beam waist necessary for high resolution. High-fidelity propagation of even a single femtosecond pulse train is a significant challenge in conventional silica fiber because of problems such as chromatic dispersion, self-phase modulation, and soliton generation. With two wavelengths, the problem is compounded by a host of nonlinear effects, including a strong stimulated Raman background (four-wave mixing [FWM]) from the silica glass itself that obscures sample signals.
To avoid these issues, the researchers designed and fabricated a hollow-core fiber that can transmit the two synchronized pulse trains required for CARS without significant distortion of their temporal or spectral properties. That’s because the two pulse trains travel in the air-filled core that has a nonlinear coefficient 1000X lower than silica. Furthermore, the group velocity dispersion (GVD) is very small (<5 fs/nm/m over the full transmission window)—hence, the temporal broadening of a ~150 fs pulse traveling over a 1 m fiber length is only a few femtoseconds and can be fully neglected.
Modifying fiber NA
For image scanning, the distal end of the fiber is attached to a four-quartered piezo tube terminating in a miniature objective. The piezo tube is resonantly driven in a typical expanding spiral pattern (see Fig. 1). Because the air core has a refractive index near 1, it must be relatively large for single-mode operation (for example, 20 µm vs. a few microns for single-mode glass fibers). The laser pulses need to be imaged down to a sub-micron spot in the tissue, which requires demagnification using a 20X microscope objective. But this means the scanning range of the fiber tip, and hence the field of view, is reduced by the same 20X demagnification factor.
Incorporating a 30-µm-diameter glass microsphere in the distal end of the fiber, which focuses the output mode to a <1 µm diameter spot, addresses the tradeoff between resolution and field of view. It allows the use of a 1.5X objective, rather than a 20X, thus producing only a minor reduction in the effective scanning field (which has a diameter of 320 µm in the present configuration). Importantly, the use of ultrashort pulses means the pulses overlap at the sample, but not in the microsphere—which avoids generating a FWM background.
Efficient signal collection
Efficiently collecting CARS and SHG signals, and then transmitting them back along the fiber to the control unit containing the filters and photodetectors, presents a challenge. Because standard hollow core limits transmission to below 700 nm, and thus prevents collection of CARS and SHG signals, the researchers designed a custom double air-clad fiber architecture with a central air core surrounded by hexagonal holes in a so-called Kagome lattice configuration (see Fig. 2). This type of multi-cell fiber lattice is known to efficiently transmit ultrashort light pulses with low loss. Light confinement inside the Kagome fiber lattice core is enabled not by a photonic bandgap, but rather by a mechanism based on inhibited coupling between the cladding modes and the guided core mode. The resulting broad transmission window makes this fiber ideal for CARS targeted at high-frequency (~2885 cm-1) CH2 vibrational bonds as found in lipids.While some dispersion occurs in the Kagome fiber during transmission, this has no appreciable impact on the return signals. Most important, the additional high-index cladding surrounded by air holes increases the effective fiber numerical aperture (NA) to ~0.6, which is enough for efficient collection of backscattered signals from the target tissue.
Signal detection, sample images
Some other system details merit a brief mention. As shown in Figure 1, the components of the distal end of the endoscope system—the hollow-core fiber, piezo scanning tube, and miniaturized objective—are enclosed in a thin metal protective housing with an outside diameter of just 4.2 mm. The distal head is thus small enough to be inserted into the user channel of many conventional endoscopes.The other end of the endoscope has been designed to record simultaneous image data based on CARS, SHG, and TPF. After these signals have been propagated back through the fiber, they are spectrally separated into three channels and filtered by a set of dichroic mirrors and bandpass filters before detection by three photomultipliers.
To evaluate the optical parameters of the endoscope, the researchers used CARS to record 2 µm polystyrene beads randomly deposited on a glass coverslip (see Fig. 3). The resulting test images show that the endoscope can obtain CARS imagery free of nonlinear background effects in the fiber. The lateral xy point spread function (PSF) for CARS images was estimated to be 1 µm in the xy plane and 7.7 µm in the z direction (from the images of 1 µm polystyrene beads).
They then acquired various images using a fresh (biopsied) sample of fatty human colon tissue, with a lipid content great enough to provide high CARS contrast at CH vibrational frequencies. The tissue was sandwiched in water between two glass coverslips with a 1-mm-thick spacer and vertically mounted in front of the endoscope probe. An acquisition time of just 0.8 s delivered images with a signal-to-noise adequate for biopsy purposes. SHG images were also acquired using the 800 nm pulses only, showing even higher xy resolution (0.8 µm).
Clinical potential
If the researchers are able to successfully implement a scheme to resolve the =CH2 and –CH3 CARS signals, a multimodal endoscope based on this design would be able to deliver intraoperative images equivalent to conventional laboratory biopsy, in real time. This capability could in turn have a substantial positive impact on patient care.
Hervé Rigneault, Ph.D. | CNRS Research Director, Institut Fresnel
Hervé Rigneault, Ph.D., is CNRS Research Director at Institut Fresnel (Marseille, France).
Alberto Lombardini, Ph.D. | Researcher, Institut Fresnel
Alberto Lombardini, Ph.D., is a researcher at Institut Fresnel (Marseille, France).
Darryl McCoy | Director of Product Marketing and Senior Product Line Manager, Coherent
Darryl McCoy is Director of Product Marketing and Senior Product Line Manager at Coherent (Santa Clara, CA).
Marco Arrigoni
Marco Arrigoni is vice president of marketing at Light Conversion (Vilnius, Lithuania). He previously served as director of marketing at Coherent (Santa Clara, CA) from 2007 through 2023.