Illumination/Microscopy: Submicron intracellular lasers promise new biological insight
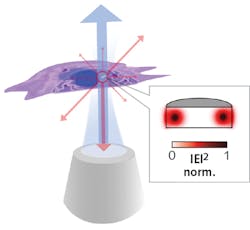
The smallest known lasers to date promise to help scientists answer “urgent questions in completely different ways” and thus better understand the mechanisms of disease, according to researchers from the School of Physics at the University of St. Andrews (Fife, Scotland) who developed them.1 With these intracellular lasers, “we can now follow individual cancer cells to understand when and how they become invasive,” says PhD student Alasdair Fikouras and Royal Society Fellow Marcel Schubert, first and second authors on the paper describing their development.
While the St. Andrews team is not the first to succeed at inserting lasers into cells, earlier lasers have been more than 1000X larger than the “nanodisks” and have required more energy to operate. These features have limited their application. By contrast, the disk-shaped nanodevices are far smaller, even, than most cell nuclei. This is important for studies requiring submicron size in each dimension—for instance, to allow migration of cells through capillaries (which have a typical diameter of 5 µm) and through epithelial layers (with pores and channels measuring 1.5 µm).
Design and fabrication
Laser miniaturization is a hot topic, especially in the pursuit of greater speed and bandwidth for communications and optical computing applications. But miniaturization of intracellular lasers has unique performance targets and tradeoffs. For instance, such lasers must work in an aqueous environment, which not only challenges their chemical stability, but also can reduce refractive index contrast between the environment and the laser material. In addition, the material, resonator, and pump should impact cell physiology only minimally. And to facilitate integration with other bioimaging platforms, such lasers should minimize tissue scattering and absorption, and perform within the typical spectral window for in vivo microscopy.
Development of the new lasers was aided by the capabilities of a new nanofabrication facility at St. Andrews. The devices are made of an aluminum gallium indium phosphide (AlGaInP) multi-quantum-well semiconductor material that is epitaxially grown. The material provides substantial optical gain to provide the brightest possible light emission, as well as color compatibility with cell biology requirements. It also enables the light sources to provide lasing thresholds 500X less than the pulse energies ordinarily for two-photon microscopy, as well as excellent spectral stability (<50 pm wavelength shift). And because the material is free of arsenic, which is common with other III–V semiconductor lasers, it is biocompatible. Featuring minute volumes (around 0.1 µm3) as well as submicron diameters (down to 700 nm), the lasers are formed by whispering-gallery mode (WGM) resonators designed to leverage concave surfaces.Intracellular operation
The inventors discovered that a variety of cells readily internalized the nanostructures, including human T-cells, which have proven too small to take up previously developed intracellular lasers, yet are important because of their role in both cancer progression and immunotherapy. In fact, the researchers say that cells can actually take up multiple lasers. Once inside a cell, the laser functions as a beacon and can report on the location of cells, or potentially even send information about local conditions within a cell (see figure).
The lasers showed no apparent effect on the behavior or viability of cells. And the WGMs showed not only low loss, but also lasing when the disk was optically pumped with sub-picojoule light pulses. The lasing wavelength depends greatly on disk size, the researchers note, which enabled them to uniquely label large numbers of cells by using nanodisks of differing diameters. Thus, they demonstrated the ability not only to produce thousands of lasers, each able to generate light of slightly different wavelengths, but also to differentiate among them.
The lasers seem especially well suited for following immune cells as they travel to inflammation sites, monitoring cancer cells as they spread through tissue, and tracking neurons, among other tasks. “Using our lasers to unambiguously track the trajectory of individual cells during these processes will provide important new insights, particularly when combined with a single cell-specific version of fluorescence activated cell sorting as well as with single-cell genomics and proteomics,” the researchers report.
REFERENCE
1. A. H. Fikouras et al., Nat. Commun., 9, 4817 (2018).
About the Author
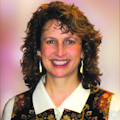
Barbara Gefvert
Editor-in-Chief, BioOptics World (2008-2020)
Barbara G. Gefvert has been a science and technology editor and writer since 1987, and served as editor in chief on multiple publications, including Sensors magazine for nearly a decade.