PHOTONICS APPLIED: SPECTROSCOPY: Planetary and deep-space applications push spectroscopy to the outer limits
With NASA's Voyager 1 spacecraft now well outside our solar system at more than 11 billion miles from the sun, it is amazing that its onboard ultraviolet spectrometer (UVS) instrument is still functional. As if the minus 31°F temperatures for which it was designed weren't bad enough, NASA turned off the heaters on the part of Voyager 1 that houses the UVS to conserve energy and extend Voyager's life into 2025. Despite its current temperature below -110°F, the Voyager 1 spectrometer—which was very active during its Jupiter and Saturn encounters—continues to transmit data.
Clearly, deep-space and planetary spectral instruments such as the spectrometers that measure our Sun and even the ALICE spectrometer from Ocean Optics (Dunedin, FL) that confirmed the presence of water ice on the moon are challenged by extreme temperatures, vibration assaults, space debris, and a host of other performance-threatening conditions, requiring scientists to develop new techniques supported by novel optical and photonic components.
Since Voyager
More than 35 years have elapsed since the launch of Voyager 1. "The Voyager UVS experiment revolutionized our understanding of the outer solar system," says Roger Yelle, professor in the Department of Planetary Sciences in the Lunar and Planetary Laboratory at The University of Arizona (UA; Tucson, AZ). "But more than that, it showed the power of UV spectroscopy—especially UV occultations."
In cooperation with the Université Paris (Paris, France) and NASA's Jet Propulsion Laboratory (JPL) at the California Institute of Technology (Pasadena, CA), the University of Arizona is using data from stellar occultations observed by the Cassini/UVIS instrument to probe the mesosphere and thermosphere of Titan—the largest moon of Saturn—at altitudes between 400 and 1400 km.1 Cassini/UVIS refers to the Ultraviolet Imaging Spectrograph (UVIS) built by the Laboratory for Atmospheric and Space Physics (LASP) at the University of Colorado (Boulder, CO) and launched into space aboard the Cassini-Huygens spacecraft by JPL in 1997.
Spectral information about Titan's atmosphere is obtained when a star is imaged above the limb of a planet. Comparison of the known spectra of the unocculted star and the spectra of the star transmitted through the atmosphere of the planet reveals the composition of the planet itself.
The Cassini/UVIS instrument consists of a telescope, a toroidal grating spectrograph, and a two-dimensional pulse counting microchannel plate detector with 1024 × 64 (spectral × spatial) "pixels," each with dimensions of 0.025 × 0.1 mm. The entrance pupil of the telescope is 20 × 20 mm and it is equipped with an off-axis parabolic mirror (22 × 33 mm) and has a focal length of 100 mm. All occultations were observed by the far-ultraviolet (112–191 nm) channel of UVIS using the low-resolution slit width of 0.15 mm, which has a field of view (FOV) of 1.5 × 60 mrad.
Spectroscopic data reveal that Titan's atmosphere is the site of complex photochemistry, forming hydrocarbon and nitrile species that play a crucial role in the formation of organic hazes observed in Titan's stratosphere (see Fig. 1). The density profiles for methane and other carbon-hydrogen compounds revealed by the spectroscopic data yield not only important information about the atmosphere of Titan as a whole but also provide broader atmospheric insight into how aerosols grow from small seed particles into large fractal aggregates.
Raman astrospectroscopy
Beyond UV spectroscopy, Stanley Michael Angel, professor and Fred M. Weissman Palmetto Chair in Chemical Ecology in the Department of Chemistry and Biochemistry at the University of South Carolina (Columbia, SC), and colleagues at the University of Hawaii (Honolulu, HI) and NASA Ames Research Center (Moffett Field, CA) are applying Raman spectroscopy to remote planetary applications.2
Just as in terrestrial handheld spectrometer measurements for explosives detection or any type of chemical/substance analysis, Raman spectroscopy can offer improved specificity for analysis of minerals and chemical compounds based on the vibrational frequencies, relative intensities, and number of bands in the spectrum of the sample. While visible, near-infrared, thermal, reflection, and emission spectroscopy suffer from broad overlapping spectra, especially in the presence of mixtures, Raman spectroscopy reduces ambiguity and lends itself to standoff measurement at distances up to hundreds of meters.
In early 2012, Angel and his team received the William F. Meggers Award from the Society for Applied Spectroscopy (Frederick, MD) for their proof-of-concept development of a spatial heterodyne interferometer-based Raman spectrometer (SHS) for planetary Raman spectroscopy.3
Unlike dispersive (grating) approaches that require large spectrographs and very narrow slits to achieve high spectral resolution, the UV SHS has only a weak coupling of resolution and throughput, so it can be small and use a wide slit to maximize throughput. The SHS—with no moving parts for robust extraterrestrial performance—measures all optical path differences in its interferogram simultaneously with an ICCD detector array, making the technique compatible with gated detection using pulsed lasers, which reject ambient background and mitigate fluorescence that could be encountered on a planetary surface with uncontrolled samples.
The European Space Agency's ExoMars mission will include an orbiter (launching in 2016) with a lander (launching in 2018) that will be the first to feature a Raman spectrometer, coupled with a laser-induced breakdown spectroscopy (LIBS) system for mineral analysis. The system will operate in either a micro-mode to look at samples crushed into fine grains (20–100 μm in size) or in macro-mode, in which a probe attached to the lander's robotic arm is extended to measure larger areas using a larger source beam diameter (a few hundred microns).
In addition to mineral identification, Raman spectroscopy is also being considered for remote detection of biological analytes such as cyanobacteria, chlorophyll, or amino acids that could indicate the presence of life beyond Earth. While studies show that a Raman system would need a demanding 16 cm-1 resolution in the spectral range from 500 to 1700 cm-1 for unambiguous identification of these biomarkers, Angel and his colleagues have demonstrated that Raman spectroscopy can detect amino acids near femtomole levels using UV excitation (see Fig. 2).Raman astrospectroscopy is also being considered for missions to Venus. Remote Raman measurements conducted at the University of Hawaii were able to identify minerals under high temperatures up to 1003 K at 9 m and under supercritical carbon-dioxide (CO2) conditions—approximately 95 atmospheres and 423 K—such as those that exist on the surface of Venus. Minerals important to Venus such as anhydrous sulfates, carbonates, and silicates were detected under both dark and ambient light conditions, demonstrating the ability of the remote Raman system to analyze surface mineralogy and identify atmospheric constituents without landing on the harsh Venusian surface.
Withstanding harsh environments
In the hot environment of the planet Mercury, continuing flybys of the Mercury Surface, Space Environment, Geochemistry, and Ranging (MESSENGER) mission show that volcanoes were involved in the formation of vast plains and that surface chemistry and mineralogy is varied, based on reflectance and high-resolution imaging data. The mechanisms of planetary formation on Mercury will be better understood through detailed mid-IR spectral and temperature analysis data from the Mercury Radiometer and Thermal-infrared Imaging Spectrometer or MERTIS, part of the European Space Agency (ESA) Mercury Planetary Orbiter mission BepiColombo scheduled for launch in 2015 with planned arrival at Mercury by 2022.4
Housing a push-broom IR grating spectrometer (TIS; 7–14 μm) and microradiometer (TIR; 7–40 μm), MERTIS consists of a series of modules for the sensor head, electronic units, and power/calibration systems with a combined 3.4 kg mass and <13 W power consumption (see Fig. 3). All modules are thermally separated for optimal performance, and the in-flight calibration routine uses sequential observation of four targets: a planet, deep space, and two onboard blackbody sources. A 45° tilted mirror between baffles and optics allows frequent views of the calibration targets (at 300 and 700 K) and both cold space and the planet Mercury, viewed through two separate baffles oriented perpendicular to each other, with a cylinder to shield the optics and electronics against incoming radiation.Its 50 mm focal-length telescope (f/2) produces a 4° field of view, enabling the TIS spectrometer to map the entire planet with a spatial resolution of 500 m. The TIS imaging spectrometer uses an uncooled microbolometer array for detection and combines a three-mirror anastigmate (TMA) with a modified Offner grating spectrometer.
"Mid-IR spectroscopy provides many different modalities for studying and mapping the composition and texture of planetary surfaces; by gathering this information on planet Mercury it is hoped to learn more about its formation and sequence of development," says Gabriele E. Arnold, staff member at the German Aerospace Center (DLR; Berlin, Germany) and the University of Münster (Münster, Germany) with more than 30 years of experience in planetary science and space flight instrumentation. "MERTIS is a state-of-the-art instrument using a modular approach. The unique challenges of MERTIS' development required new optical, optomechanical, and front-to-end electronic solutions with minimum power consumption and mass, which had to be integrated into a novel technical-engineering concept," she adds.
In addition to facing extreme weather conditions on Mercury and Venus or the everyday in-flight or orbiting hazards of deep-space debris and particle bombardment, spectrometers are also playing a role in understanding what types of optical and electronic components and materials best endure the rigors of the space environment. As part of NASA's Materials International Space Station Experiment (MISSE), two miniature BLUE-Wave spectrometers from StellarNet (Tampa, FL) were launched in 2009 on the STS-129 shuttle mission as part of the MISSE 7 instrumentation that was mounted externally to the International Space Station (ISS) to evaluate more than 700 new optical and electronic materials. The rugged spectrometers tested the optical properties of the materials as a function of exposure to atomic oxygen, UV radiation, direct sunlight, space vacuum, debris impact, and temperature extremes (see Fig. 4).Until MISSE 5, only passive material experiments limited analysis to the period before and/or after deployment; but for the first time, MISSE 7 transmitted digital data from the experiments through the ISS down to several ground stations for real-time processing.
The in situ BLUE-Wave spectral reflectivity data for the MISSE 7 revealed a better understanding of the durability of next-generation materials when exposed to space with the mission of designing future spacecraft to travel far beyond Earth's orbit. "As spectrometers are becoming more miniaturized, rugged, and lower in cost, we are seeing new and exciting applications way beyond the scope of standard lab and field research," says Jason Pierce, director of business development at StellarNet. "Who would have imagined 20 years ago when StellarNet first designed a little rugged spectrometer that one day humans would be mounting them on the Space Station to test specialized materials for exploration into the new frontier!"
REFERENCES
1. T.T. Koskinen, Icarus, 216, 507–534 (2011).
2. S. Michael Angel et al., Appl. Spectros., 66, 2, 137–150 (February 2012).
3. N.R. Gomer et al., Appl. Spectros., 65, 8, 849–857 (August 2011).
4. G.E. Arnold, et al., "Advanced mid-IR remote sensing for planetary exploration," SPIE Newsroom (Jul. 29, 2010); doi:10.1117/2.1201007.003101.
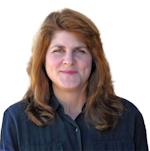
Gail Overton | Senior Editor (2004-2020)
Gail has more than 30 years of engineering, marketing, product management, and editorial experience in the photonics and optical communications industry. Before joining the staff at Laser Focus World in 2004, she held many product management and product marketing roles in the fiber-optics industry, most notably at Hughes (El Segundo, CA), GTE Labs (Waltham, MA), Corning (Corning, NY), Photon Kinetics (Beaverton, OR), and Newport Corporation (Irvine, CA). During her marketing career, Gail published articles in WDM Solutions and Sensors magazine and traveled internationally to conduct product and sales training. Gail received her BS degree in physics, with an emphasis in optics, from San Diego State University in San Diego, CA in May 1986.