‘Leap-ahead technology’ generates terahertz radiation
A team of researchers from Harvard University’s John A. Paulson School of Engineering and Applied Sciences (SEAS; Cambridge, MA), the DEVCOM Army Research Lab (Houston, TX), and DRS Daylight Solutions (San Diego, CA) recently demonstrated a compact terahertz laser that operates at room temperature and can produce 120 individual frequencies spanning the 0.25–1.3 THz region—far more than previous terahertz sources.
The terahertz frequency range, which lies in the electromagnetic spectrum between microwaves and infrared light, has remained difficult to exploit for applications because most terahertz sources are either bulky, inefficient, or rely on low-temperature devices to produce these elusive frequencies with limited tuning.
However, the team’s new terahertz laser is “a leap-ahead technology for generating terahertz radiation,” says Federico Capasso, the Robert L. Wallace Professor of Applied Physics and Vinton Hayes Senior Research Fellow in Electrical Engineering at SEAS, as well as one of the inventors of the quantum cascade laser. “Thanks to its compactness, efficiency, wide tuning range, and room-temperature operation, this laser has the potential to become a key technology to bridge the terahertz gap for applications in imaging, security, or communications.”
Even wider tuning range
Back in 2019, the Capasso Group collaborated with the Massachusetts Institute of Technology (MIT; Cambridge, MA) and the U.S. Army to create a prototype that proved terahertz frequency sources could be compact, capable of operating at room temperature, and widely tunable by combining a quantum cascade laser pump with a nitrous oxide molecular laser.
This time around, the team is using methyl fluoride—a molecule that interacts strongly with optical fields—instead of nitrous oxide (see figure). “This compound is really good at absorbing infrared and emitting terahertz,” notes Arman Amirzhan, a graduate student at SEAS. “By using methyl fluoride, which is nontoxic, we increased the efficiency and tuning range of the laser.” In fact, this work more than triples the tuning range of that prototype.
Methyl fluoride has been used as a terahertz laser gain material for almost 50 years, “but it only generates a couple of laser frequencies when pumped by a bulky carbon dioxide laser,” says Henry Everitt, the U.S. Army’s senior technologist for optical sciences. “The two innovations we report, a compact laser cavity pumped by a quantum cascade laser, combine to give methyl fluoride the ability to lase on hundreds of lines.”
As far as the basic physics concepts involved, the first is quantization of the energy level of molecules described by quantum mechanics. “The energy difference corresponding to a rotational motion of the molecule corresponds to frequencies within the terahertz region (50 GHz to 2 THz, with discrete lines roughly spaced by 50 GHz for the methyl fluoride molecule),” explains Paul Chevalier, a research associate at SEAS and the lead researcher of the team. “The energy difference corresponding to a vibration of molecules corresponds to frequencies on the order of 30 THz: corresponding to infrared light around 10 mm wavelength.”
The second concept is that the molecule has a large dipole moment, which means it strongly interacts with light. “It’s therefore able to absorb infrared light at around 10 mm wavelength—over many discrete transitions,” Chevalier adds. “And when placed in a properly designed laser cavity, it emits light in the terahertz region, and the exact frequency depends on the chosen pumped infrared frequency.”
Coolest part of this work? “For almost each frequency out of the 120 individual lines we got to lase, we’re probably the first ones to see them lasing,” Chevalier says. “This is enabled by the continuous tunability of the quantum cascade laser. There were lasers using the methyl fluoride molecule in the past, but those were using a line-tunable carbon dioxide laser, and, therefore, only a handful of those lines were able to be obtained back then.”
Biggest challenge? Not being able to see the actual light that comes out of the laser. “This makes optimization of the laser much harder because we rely on instruments that can see this terahertz light,” Chevalier says. “Those instruments have their own limitations and we have to deal with them.”
The group’s laser has the potential to be one of the most compact terahertz lasers ever designed, and they hope to further shrink its footprint.
“It can potentially become the size of a shoebox,” says Capasso. “More specifically, I think that, with proper optimization, we can make the laser itself be the size of a cube about 15 × 15 × 15 cm.”
Such a device, much smaller than a cubic foot, will allow researchers to target this frequency range for more applications—including short-range communications, short-range radar, biomedicine, and imaging.
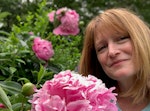
Sally Cole Johnson | Editor in Chief
Sally Cole Johnson, Laser Focus World’s editor in chief, is a science and technology journalist who specializes in physics and semiconductors. She wrote for the American Institute of Physics for more than 15 years, complexity for the Santa Fe Institute, and theoretical physics and neuroscience for the Kavli Foundation.