X-ray fluorescence-computational ghost imaging combo speeds chemical mapping
A group of Bar-Ilan University researchers in Israel recently developed a lensless imaging method to create high-resolution chemical element maps.1 Their method, based on x-ray fluorescence (XRF) and computational ghost imaging, is expected to enable chemical mapping of larger objects at higher resolutions than are possible today (see Fig. 1).
XRF determines the chemical elements within a sample by measuring fluorescence emitted from it after being excited by an x-ray source. Computational ghost imaging involves correlating two beams—one to encode a random pattern to act as a reference that never directly probes the sample, and a second beam that interacts with the sample.
The researchers tweaked the computational ghost imaging approach to measure emitted fluorescence instead of measuring transmitted radiation. This enables identification of each chemical element based on its unique emission spectrum.
Chemical mapping
“The goal of chemical mapping is to retrieve the spatial distribution of elements within an inspected sample,” says Sharon Shwartz, associate professor of physics and research team leader. “You can do this by using a lens that focuses the beam and scanning the sample with the small spot until you get the emission from every point within the sample. We don’t use lenses; instead, we use an input beam with nonuniform intensity distribution.”
They’re able to go lensless by adding a mask with nonuniform thickness to introduce nonuniform transmission that produces a nonuniform intensity distribution. “The key is we know exactly how the intensity varies at the location of the sample,” Shwartz adds. “The emitted fluorescence depends on the local intensity and distribution of the chemical element, and a detector measures intensity that is proportional to both.”
To reconstruct the spatial distribution of an element, the researchers repeat the process for different intensity patterns and by using the computational method of compressive sensing to speed up reconstruction. A chemical element map is created by combining the spatial distributions of all elements (see Fig. 2).Compressed sensing/artificial intelligence (AI) algorithms reduce measurement time, according to the group, and make the process of chemical mapping more efficient compared to standard techniques.
“With our method, the sample is irradiated by many patterns of x-ray radiation, and we measure the sample’s response to each of the patterns,” says Ph.D. student Yishay Klein. “Then, by using compressed sensing or AI, it’s possible to reconstruct the object from the known patterns and corresponding fluorescence measurements.”
The key to this approach, Klein adds, is the number of patterns required is less than the number of the pixels within the image—so its measurement time could be much shorter than for standard methods that rely on focusing the beam and raster scanning the sample.
For image reconstruction, each chemical element is done separately. The fluorescence signal from the sample includes the emission lines from each element, which can be used as a fingerprint of the element (see Fig. 3).“By using a photon-energy-resolving detector, we’re able to separate the response of each element within the object and then reconstruct—via compressed sensing or AI—the 2D image of each element,” Klein says. “The final step is to combine the images we obtained for each of the elements into one colored image where each color represents a chemical component.”
How does spatial resolution validate the researchers’ method? With standard XRF methods, “the spatial resolution is simply the width of the input focusing beam that hits the sample,” Klein says. “Since focusing within the x-ray regime is challenging, the resolution is strongly limited. In our work, focusing isn’t required. Instead, the resolution is determined by the smallest features of the mask, which spatially modulate the x-ray beam.”
To test their method, the researchers created a chemical map of an object made from iron and cobalt. Their compressive sensing algorithm reduced the number of scans by a factor of almost 10 compared to standard scanning-based techniques.
They were able to demonstrate a resolution of about 40 µm, with the possibility of improving the resolution by fabricating much smaller features through the use of state-of-the-art technologies such as x-ray lithography.
Overcoming obstacles
The two biggest challenges the researchers faced were noises on the system and finding a mask material.
“The most challenging part is dealing with noises on the system,” Klein says. “One of the elements we imaged was iron, which is a very common in almost every component of the system, like screws and holders. The detected fluorescence of iron includes not just the signal from the object but also the fluorescence from other components. In addition, some overlap exists between the different photon energies emitted from the object; among themselves, and with the photon energy of the input beam.”
In contrast to the optical regime, there are no spatial light modulators—at least not as a trivial component—and the researchers needed to implement a mask capable of modulating the input irradiation.
“The challenge was to find a mask that can induce sufficient modulation of the beam and maintain the high resolution,” Klein says. “This is because the resolution is basically determined by the feature size of the mask that induces the intensity patterns. However, mechanically, there is a tradeoff between the thickness that determines the modulation depth and the transverse dimensions of the features that determine the resolution. Fortunately, we found a simple and robust mask that complies with the resolution and modulation reequipments of our experiment. It turns out, a simple sandpaper can be very useful as a mask.”
Potential applications
By eliminating lenses and the need for focusing, the group’s method can be used to detect specific elements while “blind” to human tissues, according to Shwartz. And medical imaging, which is performed at x-ray energies where lenses aren’t practical, could benefit from this approach. It can help “increase the quality of medical imaging by boosting tissue contrast or for reducing the x-ray dose necessary to get useful images,” he adds.
Since XRF has a broad range of applications and this method makes x-ray imaging much easier and faster, “we anticipate many applications ranging from more conventional applications like materials inspection and nondestructive imaging to many cultural heritage applications,” Shwartz says. “More novel directions include new modalities for medical imaging and homeland security.”
The coolest aspect of this method, Shwartz notes, is that “it’s so simple and easy to use.”
And Klein points out that by taking advantage of simple and cheap devices such as sandpaper as a mask, they were able to demonstrate a significant improvement of the measurement time and potential improvement of the spatial resolution—this can open up a field like medical imaging to the benefits of XRF imaging.
Next, the researchers plan to extend their technique to 3D imaging.
REFERENCE
1. Y. Klein, O. Sefi, H. Schwartz, and S. Shwartz, Optica, 9, 1, 63–70 (2022).
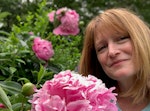
Sally Cole Johnson | Editor in Chief
Sally Cole Johnson, Laser Focus World’s editor in chief, is a science and technology journalist who specializes in physics and semiconductors. She wrote for the American Institute of Physics for more than 15 years, complexity for the Santa Fe Institute, and theoretical physics and neuroscience for the Kavli Foundation.