Single-mode semiconductor laser scales in size, power
A group of researchers led by Boubacar Kanté, an associate professor in the University of California at Berkeley’s Department of Electrical Engineering and Computer Sciences and a faculty scientist at the Materials Sciences Division of the Lawrence Berkeley National Lab, recently solved a longstanding optics challenge.
By creating a scalable laser cavity out of a semiconductor membrane perforated with tiny, evenly spaced holes, which they call “Berkeley Surface Emitting Lasers” or BerkSELs, Kanté’s group (see Fig. 1) was able to systemically eliminate higher-order modes from lasing.
“There may be manufacturing challenges ahead, but the 60-year-old roadblock of how to increase the power of surface-emitting lasers is now gone,” says Kanté. “In the future, manufacturers won’t need hundreds of lasers—they can be replaced by one laser that will emit enough power.”BerkSEL design
The BerkSEL design enables single-mode light emission as it passes through tiny lithography-etched holes in a 200-nm-thick membrane made out of indium gallium arsenide phosphide (InGaAsP; see Fig. 2). The holes serve as Dirac points—a topological feature of 2D materials based on the linear dispersion of energy (see Fig. 3).They selected the semiconductor material and the dimensions of the structure to enable lasing at a telecommunications wavelength, although BerkSELs can emit different target wavelengths by tweaking design specifications, such as hole size and semiconductor material.
Since the invention of the laser, optics researchers have dealt with coherent, single-wavelength directional light starting to break down as the size of the laser cavity increases by turning to external mechanisms like waveguides to amplify the light. And lots of power requires a long waveguide.
“The best way to increase laser power is via surfaces because a surface’s area is the square of its linear dimension,” Kanté says. “If you increase the area, power goes up much faster than with a waveguide.”
Kanté is fascinated by electromagnetism in general, and came up with and demonstrated the concept of a topological laser—based on the quantum Hall effect for light—in 2017. It turns out, this discovery has a role to play enabling BerkSELs.
Dropping the refractive index to zero
Kanté’s group showed a cavity’s free spectral range (think lots of modes in the cavity)—the distance between modes—is a complex number, meaning the distance has “real but also imaginary parts.” Currently, as the size of a laser cavity increases, modes become closer in frequency and can’t be separated. If one mode lases, others also start to lase as the cavity size grows.
But they figured out a way to drop the refractive index of the laser to zero, so all elements at the surface of the laser are in phase. “This doesn’t happen in any existing laser,” he points out. “Imagine preventing all other modes from lasing and bumping the entire power of the cavity into a single mode. You immediately see how powerful such a laser can be.”
The optical phase between two points is the refractive index between the points multiplied by the distance between the points. “If the refractive index is zero, the phase is the same everywhere and all resonators are contributing—this makes the system incredibly efficient,” Kanté says.
Until now, this wasn’t possible in vertical-cavity surface-emitting lasers (VCSELs) because their aperture is on the order of microns and couldn’t be made larger. “Our work shows you can make the aperture as large as you’d like. Since the device is planar, manufacturing will be easily figured out,” he adds.
Scaling
How is Kanté’s earlier work inventing a topological laser involved? All existing surface-emitting lasers emit light only from the middle of their cavity, and it’s from the fundamental mode of potential wells. “Energy will be at the center, with zero at the boundary,” he says. “In BerkSELs, light comes from everywhere and there’s no space left for any other mode to come into play. Higher-order modes lasing is forbidden by the physics of this cavity.”
One amazing aspect of this work is “no one has predicted or observed this type of mode within any potential well,” Kanté says. “We discovered an ‘open-Dirac’ potential that enables scaling for these lasers. To make a system scalable, it needs some type of losses. Open means there are some losses in the system so the higher-order modes get dumped into the lossy modes. This will have a lot applications in physics, especially for the wave physics community.”
Importantly, the group discovered a new solution in a potential well. “This is a surprise for not only the laser community, but also the wave physics community,” he says. “The biggest challenge in quantum science today is scaling—how can we increase the number of qubits while maintaining the coherence of a system? It’s the same scaling problem lasers have, because increasing the size while maintaining the cavity in a single mode means increasing the size and scaling while maintaining coherence.”
Kanté is currently working on proposals to demonstrate systems based on this device. “It can be used for LiDARs, for example,” he says. “LiDARs depend on the power of the source, and now that it can be increased, you can make a very compact device to use for various LiDAR systems. With very tiny but powerful lasers, you can put them on any small robot you can think of. We now need to translate this fundamental discovery into a technology integrated into systems.”
While it may take a few years to transition this technology to devices, “it should be fairly fast, because the technology is relatively simple and compatible with current nanomanufacturing,” he adds.
RELATED READING
B. Bahari et al., Science, 358, 6363, 636–640 (Oct. 12, 2017); https://doi.org/10.1126/science.aao4551.
T. Wogan, Physics World online (Oct. 27, 2017); https://physicsworld.com/a/physicists-create-first-topological-laser.
R. Contractor et al., Nature (2022); https://doi.org/10.1038/s41586-022-05021-4.
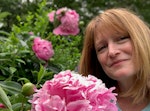
Sally Cole Johnson | Editor in Chief
Sally Cole Johnson, Laser Focus World’s editor in chief, is a science and technology journalist who specializes in physics and semiconductors. She wrote for the American Institute of Physics for more than 15 years, complexity for the Santa Fe Institute, and theoretical physics and neuroscience for the Kavli Foundation.