What goes into building amplifiers for an attosecond x-ray free-electron source?
The Center for Free Electron (CFEL) Science’s Ultrafast Optics and X-Rays (UFOX) Group at the German Electron Synchrotron (DESY) is building a new ultrafast laser x-ray source to study electronic motion within molecules to help explore complex chemical and biological reactions.
The primary goal of this project, known as “AXSIS: Frontiers in Attosecond X-Ray Science, Imaging, and Spectroscopy,” is to develop an attosecond x-ray free-electron source based on coherent inverse Compton scattering—in which a charged particle transfers part of its energy to a photon.
A second goal is to miniaturize the accelerator via terahertz radiation for acceleration. Compared to conventional accelerators, which are based on radio waves, the accelerator components also need to be built on a much smaller scale.
The AXSIS system requires several 100 millijoules- to joule-class laser amplifiers with a nanosecond to femtosecond (fs) pulse width to operate.
For the group’s design, their amplifier systems are based on cryogenically cooled ytterbium-doped yttrium lithium fluoride (Yb:YLF) and ytterbium-doped yttrium aluminum garnet (Yb:YAG) lasers for easier scaling of the operating repetition rate of the system. This is necessary to generate the required THz energies to power an electron accelerator and finally produce the x-ray flux needed for imaging.
These Yb:YAG/YLF-based 1 µm amplifier systems rely on the chirped-pulse amplification concept—honored with the 2018 Nobel Prize in Physics—and require versatile low-energy seed sources for optimum operation.
“The central wavelength of these seed lasers should ideally match the gain maxima of cryogenic Yb:YLF and Yb:YAG systems, which have strong emission peaks located around 995 nm, 1017 nm, and 1030 nm,” says Ümit Demirbas, a senior laser scientist in the UFOX Group. “As a result, we’re also investigating several alternative laser gain media as seeders, including Yb:fiber, Yb:YLF, Yb:vanadate, and Cr:LiSAF (chromium-doped lithium strontium aluminum fluoride/colquirilite) lasers.”
Exploring limits of Yb:YLF wavelength tuning and femtosecond pulse generation
The researchers are exploring the limits of Yb:YLF in terms of wavelength tuning and femtosecond-pulse generation to better understand its capability as a versatile seeder for cryogenic Yb-based amplifiers (see figure).
To push the laser material to its limits, “we first use a low-loss laser crystal with quite low passive losses (of only around 0.0005 cm-1),” says Demirbas. “Today, Yb:YLF crystals can be grown with very low optical loss up to Yb dopings of 10%, which also make it the material of choice for laser-cooling applications.”
To optimize wavelength tuning behavior, they use a specially designed off-surface optical axis crystal quartz birefringent filter for tuning. “Compared to regular on-surface optical axis birefringent filters, off-surface optical axis offers many advantages, such as a much larger side-band rejection, which is required to successfully tune the laser wavelength to the edges of the gain spectrum.”
For modelocking, the group uses a semiconductor saturable absorber mirror (SESAM) to initiate pulsing and negative dispersion mirrors for soliton pulse shaping.
“Our work with room-temperature Yb:YLF shows that low-loss crystals can achieve slope efficiencies approaching 80% in Yb:YLF lasers—even while pumping with low-brightness multimode diodes,” says Demirbas. “And we’ve achieved a record continuous wave tuning range covering the 993- to 1110-nm region.”
Thanks to modelocking, the group achieves pulses as short as 40 fs around a central wavelength of 1040 nm. They believe these pulse widths can be reduced to a sub-25-fs duration via optimization of the overall dispersion of the cavity.
“The central wavelength of the fs pulses was tuned over the 1019- to 1047-nm range to obtain sub-500-fs-level pulses,” Demirbas says. “We also demonstrated 380 fs pulses with up to 1.85 W average power, at an optical-to-optical conversion efficiency above 20%.”
One of the most significant aspects of this work is the ability to tune the laser wavelength up to 1100 nm—a wavelength far off the gain peak of Yb:YLF crystals.
The laser gain within this wavelength region is more than 100x smaller than the gain at the peak of the emission around 1025 nm. “This makes using off-surface optical axis birefringent filters with large sideband rejection and low-loss Yb:YLF crystals crucial to get this performance,” Demirbas explains. “The expected quantum-limited timing jitter of such oscillators is within the attosecond range, demonstrating the potential of these systems as ultralow-jitter oscillators.”
Generating terahertz light
What’s next? “Our group is working on cryogenic Yb:YLF amplifier systems with pulse energies up to the joule level at a repetition rate of 1 kHz,” says Mikhail Pergament, who leads the laser development efforts of the UFOX Group. “Using optimized seed sources, we hope to reach an output pulse width down to 250 fs from these amplifiers, which can’t be achieved using Yb:YAG-based technologies.”
The researchers plan to use this short-pulse amplifier system to efficiently generate terahertz light within the 100- to 500-GHz range, which is necessary for their electron accelerators, as well as coherent attosecond x-ray generation.
“We’ll also pursue high-energy pulse compression,” says Franz Kärtner, leader of the UFOX Group and a physics professor at Hamburg University. “Due to its exceptional high beam quality, the Yb:YLF amplifier is also an excellent source for pumping of optical parametric amplifiers (OPA) and optical parametric chirped pulse amplifiers (OPCPA). And the multi-GHz repetition rate Yb:YLF oscillators will be further explored as potential ultralow-timing-jitter oscillators for photonic microwave generation and other applications.”
RELATED READING
F. X. Kärtner et al., Nucl. Instrum. Methods. Phys. Res. B, 829, 24–29 (2016).
U. Demirbas et al., Opt. Lett., 47, 933–936 (2022).
U. Demirbas et al., Appl. Opt., 61, 13, 3702–3710 (2022).
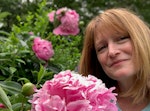
Sally Cole Johnson | Editor in Chief
Sally Cole Johnson, Laser Focus World’s editor in chief, is a science and technology journalist who specializes in physics and semiconductors. She wrote for the American Institute of Physics for more than 15 years, complexity for the Santa Fe Institute, and theoretical physics and neuroscience for the Kavli Foundation.