INDUSTRIAL LASERS: Pulse control in high-power UV laser enables new micromachining options
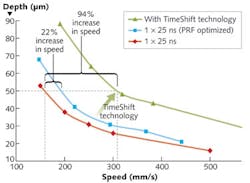
Mobile consumer electronic devices such as smart phones and tablets represent one of the largest and fastest evolving segments in the global manufacturing industry.1 Continual technology development efforts are required to build up advanced precision laser manufacturing processes for key components such as semiconductor chips, microelectronic packages, touch-screen displays, and printed circuit boards. The current generation of diode-pumped solid-state (DPSS) lasers has, in many cases, played an enabling role with new techniques that make it possible to produce sophisticated products at mass-market costs. However, just as the mobile devices grow more capable and complex, so must manufacturing processes evolve.
Given the variety of materials and processes that lasers address in this manufacturing space, it can be difficult to choose laser characteristics enabling broad advances in high-quality, high-speed micromachining. Such processes are strongly influenced by a number of laser parameters, including wavelength, pulsewidth, average power, beam quality (M2), pulse repetition frequency (PRF), and pulse-to-pulse energy stability. And, with the advent of pulse shaping and pulse splitting, the list of ingredients in an optimized process recipe grows longer.
Lasers having shorter wavelength, shorter pulsewidth, and low M2 benefit micromachining processes by creating a tightly focused spot and minimizing heat affected zone (HAZ). In general, shorter wavelengths are strongly absorbed by many materials, making them suitable for machining a wide variety of organics, semiconductors, ceramics, and metals. High energy absorption, as well as high on-target irradiance levels created by short pulses, vaporizes material quickly, reducing HAZ and charring. The small, focused beam spot enables machining smaller features with higher precision. Higher power, higher PRF, pulse-shaping, and pulse-splitting capabilities all can contribute to improved micromachining throughput. And consistent higher pulse-to-pulse stability ensures process repeatability and helps achieve higher process yield.
Although UV Q-switched (Q-SW) DPSS lasers have met manufacturing demands, they have limitations in achieving higher speeds. A common approach to increase the processing speed is boosting the PRF of the laser while holding other process parameters fixed. However, for a typical Q-SW DPSS laser, this is really not achievable. For these lasers, the average power and pulse energy decrease quite rapidly as the PRF increases. Also, the laser pulsewidth and pulse-to-pulse energy variability tend to increase significantly.
Since the variation in these laser parameters affects the micromachining speed, feature size, and accuracy achieved, simply increasing PRF is often not enough to maintain process results as we attempt to scale up throughput. The real and sought-after solution to overcoming these limitations is a laser that not only maintains high average power at the higher PRFs but also continues to deliver shorter pulsewidths with low pulse-to-pulse energy variation, pulse-shaping, and pulse-splitting capabilities, to achieve both higher throughput and cleaner ablation results.
To meet the need for such laser technology, we developed a UV hybrid fiber laser, the Quasar 355-40, which combines higher power at higher PRF. It also features a software-adjustable technology called TimeShift for setting a wide range of pulse energies and pulsewidths. This technology also gives the process engineer the ability to create pulse intensity waveforms in the time domain through techniques such as pulse shaping, pulse splitting, and burst-mode operation. The laser has an output power of >40 W of 355 nm wavelength at 250 kHz PRF and eliminates the dependence of pulsewidth on PRF, making it possible to hold all characteristics of the laser output steady as throughput is scaled up by increasing the repetition rate.
The combination of higher power at high PRF, independently adjustable pulsewidth, and pulse-manipulation capabilities are proving beneficial for micromachining of various microelectronics materials. Here, we present initial results achieved in silicon, glass, and printed circuit board (PCB) materials.
Silicon dicing in semiconductor fabrication
In microelectronics manufacturing, hundreds or thousands of integrated circuits or other micro-devices are fabricated on a single semiconductor (silicon) wafer and are finally separated or diced into individual chips. Laser dicing of silicon wafers has been in use for several years as an alternative to conventional dicing with a precision rotary saw. As the wafers become thinner and as lasers become more reliable and powerful, the advantages over saw-based dicing increase dramatically.
Moreover, with the advanced features now available, it becomes easy to manage thermal loading in the material while maintaining high micromachining throughput. This is especially important in silicon dicing, where thermal effects can either lead to micro-cracks along the diced chip edges (which decrease mechanical die strength) or possibly damage the electronic devices themselves.
An alternative to through-dicing is to create a laser-ablated groove or scribe line in the wafer "streets" between chips. This is done either as a first step in a two-step scribe-and-cleave process or as a step before saw dicing when delicate or brittle materials are present. At our applications lab, we demonstrated scribing of ~100-μm-thick polished single-crystal (<100>) silicon wafers using the Quasar 355-40 laser.
Very high scribing speeds were achieved, while avoiding any thermal damage to the material. In a first experiment, we compared the scribe depth obtained with the laser operating at three different conditions: a) a single pulse per focal spot location, having 25 ns pulsewidth at 200 kHz PRF; b) using TimeShift to maintain that single 25 ns pulse at higher PRF of 300 kHz; and c) a burst of pulses using the TimeShift pulse-splitting feature (10 sub-pulses of 5 ns, separated by 10 ns), with bursts repeated at 255 kHz frequency.
In Fig. 1, the curve for single 25 ns pulses at 200 kHz establishes the basic trend that as scribe speed increases the scribe depth decreases. Using the high UV output power from the laser, these parameters deliver impressive depth vs. speed results. To increase scribe speed while maintaining a given depth, the TimeShift feature was used to generate the single 25 ns pulse at even higher PRF of 300 kHz. We observed a 22% increase in the scribe speed for a 50-μm-deep scribe. Finally, we further optimized the process by using pulse splitting to achieve a 94% increase in the speed over a single 25 ns pulse-scribing condition for a 50-μm-deep scribe. We believe additional improvement is possible by optimizing features such as adjustable pulsewidth and pulse shape.
In a second experiment, we investigated possibilities for increasing the scribe depth obtained at various work-surface fluences using the pulse-splitting capability. Varying the pulse energy, we generated laser scribes at various fluence levels, while holding scanning speed at 250 mm/s and PRF at 200 kHz. One set of data was collected with a pulsewidth of 25 ns, while in a second set we split the pulse into five 5-ns sub-pulses separated by 10 ns.
Figure 2 shows the clear advantage of using sub-pulse burst micromachining over single-pulse machining. By splitting the energy available in a single 25 ns pulse into five sub-pulses, an increase in ablation depth between 52 and 77% was obtained, depending on fluence level. We note that this is consistent with other studies finding enhanced material-removal rates for burst-mode ablation.2-5
We also compared the visual appearance of debris and HAZ for the two cases. Figure 3 shows representative top-view images of the scribes. Burst-mode scribes demonstrate high ablation quality with less loose debris on the top surface, despite the fact that the depth achieved was 25% greater than that achieved using single pulses. We believe that the burst-mode process creates gentler ablation with each lower-energy sub-pulse, transferring less heat into the material compared to the single-pulse case.
Glass cutting in flat-panel displays
In the display manufacturing process, touch-screen and LCD modules mostly require straight cuts for singulating small pieces of glass from large production panels. As glass substrates used in consumer electronics displays continue to become thinner and harder, laser glass-machining tools are showing great potential for providing high-quality cuts and high throughput while mitigating yield losses associated with the conventional mechanical scribe-and-cleave process.
In our applications lab studies, the Quasar was used to make clean, quality linear cuts at high throughput on 0.55-mm-thick display glass. Control of pulse temporal characteristics facilitated tailoring of the individual laser pulses to reduce thermal loading in the material, which resulted in good quality cuts at faster scanning speeds, improving overall processing throughput.
We achieved linear cutting speed of 1 m/s, highly desired in the manufacturing process. Figure 4a shows a top view of the cut edge, seen from the front side of the glass. Clean edge quality is evident, with very minimal chipping (<10 μm). Figure 4b shows a side view of the cut edge, where we observed roughness within the acceptable range of typical manufacturing requirements.
PCB processing
In manufacturing consumer electronic devices, lasers routinely perform a variety of machining processes on PCB materials. These processes include blind- or through-via drilling, depaneling, profiling, laser direct patterning, repair, trimming, marking, and skiving. PCBs may comprise a variety of materials, layers, and thicknesses, and each material type and process is likely to have specific requirements for optimal laser parameters; consequently, flexibility in tailoring laser pulses is important.
For example, a typical blind-via drilling application involves clean removal of thin resin material to expose an underlying copper layer. Figure 5 shows laser-drilled 60-μm-diameter blind vias in PCB material comprising a 50-μm-thick resin layered over copper. In this particular material, shorter pulsewidths (<20 ns) are better suited to achieve cleaner resin removal, fully exposing undamaged copper at the bottom of vias. Also, high power at higher PRF is desirable to achieve higher throughput.
With conventional Q-SW UV DPSS lasers, throughput scaling is limited by insufficient pulse energy at high PRF. If highest power is restricted to lower PRFs, maximum throughput is achieved only with the use of a split-beam/parallel processing approach—something that is often undesirable from a system cost and complexity standpoint. High power and shorter pulsewidths are available with the Quasar laser at higher PRFs, allowing for throughput maximization by operating at the higher PRF and lowering the exposure time.
The result is clean blind vias with minimal damage or oxidation to the exposed copper surface and minimal sidewall taper. Both of these process results are important for assuring good adhesion of the subsequent copper plating layer. Depending on the via diameter and resin thickness, throughputs approaching 4000 holes/s are possible.
In summary, results for our lab tests demonstrated that with proper parameter optimization, high quality and high throughput can be achieved with a UV nanosecond-pulsed laser source, extending the capabilities of laser micromachining processes to meet the manufacturing challenges of consumer electronics products.
REFERENCES
1. ReportLinker web site, "Global Consumer Electronics Market Outlook 2015"; http://bit.ly/YHkfkE.
2. P.R. Herman, R. Marjoribanks, and A. Oettl, "Burst-Ultrafast Laser Machining Method," US Patent No. 6,522,301 (2003).
3. B.R. Campbell, T.M. Lehecka, J.G. Thomas, and V.V. Semak, "A Study of material removal rates using the double pulse format with nanosecond pulse laser on metals," Proc. ICALEO 2008, 181–186.
4. W. Hu, Y.C. Shin, and G. King, "Modeling of multi-burst mode pico-second laser ablation for improved material removal rate," Appl. Phys. A, 98, 2, 407–415 (February 2010).
5. R. Knappe, H. Haloui, A. Seifert, A. Weis, and A. Nebel, "Scaling ablation rates for picosecond lasers using burst micromachining," Proc. SPIE, Laser-based Micro- and Nanopackaging and Assembly IV, 7585 (2010).
Rajesh Patel | Independent Consultant
Rajesh Patel, now an independent consultant in laser applications, was with MKS Spectra-Physics from 2006 through 2018, with his most recent role there being Director of Strategic Marketing and Applications Engineering.
Jim Bovatsek | Applications Engineering Manager
Jim Bovatsek is an Applications Engineering Manager at Spectra-Physics (MKS Instruments; Santa Clara, CA).