A team of physicists led by MITRE’s Gerry Gilbert, a theoretical physicist and quantum expert, are working on a quantum moonshot: they’re on a mission to build a scalable universal quantum computer.
This moonshot is a collaborative effort already in its third year, and includes a partnership with experimental physicists from Dirk Englund’s team at MIT and Matt Eichenfield’s team at Sandia National Labs.
A universal quantum computer is capable of “solving any quantum-computable problem,” says Gilbert. “We’re aiming for the big prize, the kind of machine that can, in principle, solve any quantum computable problem—breaking codes, solving differential equations faster, etc.”
Choose your qubits wisely
The first decision you need to make when building a quantum computer is what physical instantiation of quantum bits (qubits; a two-level quantum system) you’re going to work with. “Our choice was a combination of two, a hybrid,” Gilbert says. “For our quantum moonshot approach, a qubit is a combination of the quantum mechanical state of an individual photon, a particle of light, and the spin state of an artificial atom within a solid-state material defect.”
Each photon is characterized by a quantum mechanical state. And you can organize the physics, the dynamics of photons, to be one or the other of two possible conditions when you measure them. “Once you’ve done this, you have a qubit in principle,” he says. “This qubit, first of all, needs to be a bit—two possible ways of being measured—either up or down or 0 or 1. It also needs to be characterized by the dynamics of quantum mechanics and, by nature, photons are.”
The other half is more complex. “We use microscopic pieces of highly purified embedded diamond with defects,” Gilbert explains. “Where there should be a nitrogen atom, there isn’t one—it’s a ‘nitrogen vacancy.’ Think of it as a little hole in the diamond matrix. Within that cavity are quantum mechanical states that act as though they comprise an atom. But there’s nothing there; no atom. These quantum states are constrained by the surrounding material, the nondefect part of the diamond, to exhibit physical dynamics as if they were in an atom. We call these artificial atoms.”
These artificial atoms have an intrinsic spin, a quantum mechanical angular momentum. “The quantum mechanical state of that spin is the other part of our quantum bit,” he says.
So the quantum mechanical states of the photon and the spin states of these defects make up the quantum bits. “One serves as memory and the photons are used to transfer around the chip the strange but essential quantum mechanical correlation called entanglement, which is like gasoline for a car. You need entanglement for the quantum computer to work and get around. It gets around by being ‘carried’ on photons,” Gilbert says.
Controlling quantum mechanical states/scaling fabrication
To control the quantum mechanical states of the photonic part of their qubit, the scientists designed microscopic-size devices called cantilevers on their chip to enable them to modulate the states of the light that comprises the photonic part of the qubits.
The operation of such devices typically dissipates a lot of heat, which is bad for qubits, so you want to keep them cold. “Hot environments mean things move around, and the more they move around within the environment the more they bump into the qubits—and the state of the qubit becomes altered or decoherent,” Gilbert explains. “We don’t want this.”
They came up with a novel approach using CMOS circuits, which can be fabricated by foundries on an industrial scale. “This is an important development, because our approach is compatible with large-scale fabrication,” Gilbert points out. “It’s incredibly important for the scalability issue and, instead of relatively high heating caused by thermo-optical control, we apply a tiny voltage to a piezoelectric device on our chip. It changes its shape when the voltage is applied, and allows the modulation of the photons, which is what we want.”
By leveraging advanced semiconductor manufacturing, the team developed a new type of optical chip to connect quantum information across individual photons and individual electrons. To route photons via optical wires between spin memories, which serve as the transistor and memory equivalent in classical computers, these chips had to be developed to operate within 1°C of absolute zero.
Unlike the thermo-optical control approach, which dissipates of order of 10 mW, the group’s new approach dissipates of order nanowatts, which is a tremendous power and heat dissipation decrease. “It’s a huge advance,” says Gilbert. “Thermo-optically controlled switching speed is typically measured in microseconds. In our case, the switching speed is measured in tens of nanoseconds, which is an improvement of a factor of hundreds to thousands, depending on the details, in switching speed in our chips. We think we’ve found a unique solution that will be sufficient for us to achieve a scalable universal quantum computer. There’s a lot more to it, but this is an essential piece.”Error correction
To achieve scalability, it’s essential to reduce overhead of bits when making a quantum computer. One of the biggest challenges involved is to become fault-tolerant, which can be achieved through error correction.
“Quantum bits get bumped into by everything around them, change their state as a result, and become decoherent,” says Gilbert. “You want to prevent this and/or correct it. You can try to prevent it from happening or attempt to fix it via error correction, or both. A fault-tolerant machine is one that, despite errors, continues to work. This can be achieved if the rate at which the errors occur is smaller than the fault-tolerance threshold, but error correction is costly in terms of bits. It takes a lot of redundancy of qubits to achieve this, so you want to do it with as little overhead of bits as possible. Everyone who builds a quantum computer has to deal with this.”
In the MITRE team’s case, qubits are part photon, part solid-state. The photonics part moves around the chip so, unlike other approaches, they aren’t required to design protocols that only work between nearest-neighbor qubits. “It’s not a constraint in our case,” says Gilbert. “We believe this is a theoretical and practical advantage of our approach.”
Quirky surprises along the way
Everything about quantum mechanics is surprising and, as Gilbert points out, for most people it’s also counterintuitive.
“Quantum mechanics is the best understood thing in human existence in terms of precision and measurement,” he says. “But there are really strange things about it that are difficult to understand because uniquely quantum mechanical effects are mostly restricted to the domain of the very, very small. If you were the size of an atom and it was your world, the quantum mechanical effect would be everywhere all the time and it might be more intuitive to humans.”
In our daily activities, quantum mechanics are at play but highly suppressed. “Mathematically, quantum mechanical effects are exponentially suppressed,” Gilbert says. “So we don’t walk through walls, but in the regime of the very small, objects at the scale of atoms—such as electrons—can and do ‘walk through walls.’ They go through potential barriers via quantum-state mechanical tunneling. It’s difficult to grasp this based on our ordinary experience. We understand mathematically what’s happening, but even for physicists who use the mathematics every day, since no one has directly experienced it, it’s indeed counterintuitive.”
Gilbert likens working with quantum mechanics as akin to the pleasure of experiencing beautiful music, and says “you could call it quirky, but it’s our blinders we have because of our size that makes quantum mechanics appear as weird as it does.”
A unique opportunity
The MITRE-led quantum moonshot is a unique opportunity because it was designed so the physicists won’t need to worry about frequently applying for funding on a regular basis.
“We have the resources and the time we need to fully focus on this problem—the moonshot is an incredible gift we’ve been given,” says Gilbert. “We’re very excited about this and believe in our hybrid approach—a solid-state and photonic qubit. We think it has the right features within the quantum mechanical world to make a quantum computer.”
As far as whether or not quantum computing is “hype,” Gilbert is quick to point out that while there’s a lot of misinformation out there, he’s devoted 20 years of his life to this endeavor, which would be crazy to do if it was “hype” or didn’t have a chance of succeeding.
“We don’t know how close we are, but we do know what the challenges are, and potentially anything is possible,” he says. “Brilliant breakthroughs could be made that would enable us to have a quantum computer sooner than you’d think. But it’s an open-ended question of when it will happen. But we know of no reason based on physics or engineering why it cannot be done, so it’s absolutely not out of reach. We know what the challenges are and need to solve them.”
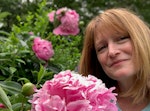
Sally Cole Johnson | Editor in Chief
Sally Cole Johnson, Laser Focus World’s editor in chief, is a science and technology journalist who specializes in physics and semiconductors. She wrote for the American Institute of Physics for more than 15 years, complexity for the Santa Fe Institute, and theoretical physics and neuroscience for the Kavli Foundation.