In a quantum nanophotonics breakthrough, a new type of polaritonic nanocavities is redefining the limits of light confinement. A team of physicists from Bar-Ilan University in Israel and the Institute for Photonic Sciences (ICFO) in Spain created nanocavities with a subwavelength volume (smaller than 100 × 100 nm2 in area and merely 3 nm thick), which can confine light for significantly longer durations than previously possible (see video).
The team’s work provides a new solution in a long-standing quest to find ways to cram photons into increasingly small volumes. When a photon gets crammed into a cavity much smaller than a wavelength, it effectively becomes more concentrated, which enhances interactions with electrons and amplifies quantum processes within the cavity.
“This all started as an accidental observation,” says Hanan Herzig Sheinfux, an assistant professor at Bar-Ilan University, who was at the time working Professor Frank Koppens at ICFO. “We were working on a completely different project, routinely using nearfield optical microscopy as a characterization tool to see if some process was working or not. I was actually going over the results of one of the routine characterization scans when I noticed something odd.”
This “hmmm…that’s weird” moment was triggered by seeing a stronger-than-anticipated reflection at one of the interfaces, which made Herzig Sheinfux wonder if it could lead to something useful.
As a side project, the team decided to recreate these strong reflections, combine several reflections, and confine the light within a small volume. “We didn’t expect to see much at first,” Herzig Sheinfux says. “I was expecting to get some preliminary indication the idea works and maybe pass the work to a young Ph.D. student as a nice and simple project.”
But the results indicated the reflections were much stronger than anticipated, and the photon confinement was far better than anticipated as well.
“Our results are opening the door to new quantum electrodynamics experiments, exploiting the power of ‘weird’ concepts like vacuum fluctuations,” says Herzig Sheinfux. “These experiments and this work can have some practical applications, but the physics involved is astoundingly beautiful. There’s some very deep and thought-provoking concepts hidden within what seems to be the relatively simple cavity designs. It’s like a work of art—except it’s made by nature, not Mozart or Picasso.”
New polaritonic nanocavity confinement technique
The team’s technique taps an exotic form of light: hyperbolic-phonon-polaritons. Hyperbolic-phonon-polaritons are electromagnetic excitations that occur within the two-dimensional (2D) hexagonal boron nitride (hBN) material, a.k.a. white graphene, which forms the cavity. And it uses a new and indirect confinement mechanism: nanocavities are created by drilling nanoscale holes into a gold substrate with the 2- to 3-nm precision of a helium-focused ion beam microscope.
After the holes are drilled, hBN flakes are placed atop these nanometric holes within a gold substate to support electromagnetic excitations (the hyperbolic-photon polaritons). “It’s a lot more complicated than it sounds,” Herzig Sheinfux says. “For example, the holes in the gold must be exceptionally precise—10 to 20 atoms in the wrong place would spoil the response of the cavity completely.”
Essentially, cavities are created by changing the environment of the polaritons as they travel through a flake of hBN. “A sharp change of this environment implies a sharp change in polaritonic behavior, which causes the polaritons clog up and be confined within a small area,” says Herzig Sheinfux. “Think of it as roughly akin to a traffic jam that begins because of car congestion when a two-lane expressway is reduced to a one-lane expressway.”
Hyperbolic-photon polaritons can be confined to extremely small volumes. When polaritons pass above the edge of the metal, they catch a strong reflection—and get confined. The team’s approach avoids shaping hBN directly to preserve its pristine quality and enable highly confined and low absorption photons within the cavity.
The biggest obstacle in their work—and the origin of the team’s second discovery—occurred when Herzig Sheinfux realized the cavities weren’t merely responding really strongly, they were in fact responding more strongly than should be possible. “Based on the theory we started with, the cavities were supposed to function half as well as they actually did,” he says. “This stumped us because experiments don’t normally outperform theory, and because all of the theoretical explanations we had didn’t make sense.”
They eventually puzzled out that the high-performance level of these cavities is actually related to intricate multimodal interference effects, which are extremely complex. “It involved lots of hard work to get to this point, and a lot of explanations that looked promising ended up making no sense,” says Herzig Sheinfux.
Once the team found their working hypothesis, everything clicked into place. “It was satisfying to find this elegant solution—and it’s the type of experience that made me certain I wanted to become a physicist in the first place,” Herzig Sheinfux adds.
Harness quantum fluctuations within nanocavities?
Nanocavities are used in physics to enhance light-matter interactions. But previous generations of nanocavities were plagued by significant amounts of absorption, which prevented some of the most exotic quantum electrodynamics from being achieved.
“With our new hypercavities as a platform, we hope to be able to harness quantum fluctuations that occur within empty cavities,” says Herzig Sheinfux. “There’s no real vacuum within our quantum universe—there’s some energy even inside what’s supposed to be an empty box and we hope to observe the fluctuations induced by this energy, to measure their effects, and maybe to use them to enhance various processes. Finding a way to do it is a challenging task, but it’s the top priority for us now.”
FURTHER READING
H. Herzig Sheinfux et al., Nat. Mater. (2024); https://doi.org/10.1038/s41563-023-01785-w.
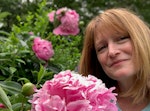
Sally Cole Johnson | Editor in Chief
Sally Cole Johnson, Laser Focus World’s editor in chief, is a science and technology journalist who specializes in physics and semiconductors. She wrote for the American Institute of Physics for more than 15 years, complexity for the Santa Fe Institute, and theoretical physics and neuroscience for the Kavli Foundation.