Quantum interference overcomes photoexcitation annihilation
A team of Purdue University (West Lafayette, IN) and Northwestern University (Evanston, IL) researchers experimentally demonstrated control of annihilation by quantum interference—proving photoexcitation is even more intriguingly complex than we imagined (see video).
Photoexcitation occurs when a photon interacts with a material and causes its atoms to change their quantum state (see Fig. 1).
“When photons are absorbed by molecules, it causes their electrons to jump to higher energy levels,” says Libai Huang, a professor of physical chemistry at Purdue University. “These excited electrons and the associated vacancies they leave behind bind together into something known as excitons, which are fascinating because they carry energy within the molecules.”
In photosynthesis, exciton migration plays a vital role in the initial stages of light energy conversion. These excitons migrate through a network of molecules, efficiently transferring the captured energy to reaction centers where chemical reactions occur.
This energy transfer process is essential for powering the biochemical reactions that drive the synthesis of sugars and other molecules vital for plant growth. Similarly, in solar cells, exciton migration is crucial for efficient energy harvesting.
“But there’s a persistent belief that excitons annihilate each other when they try to come into contact, which limits their densities and mobility,” says Huang. “This mutual annihilation, in turn, has long been seen as a bottleneck that impedes the efficiency of solar cells and the natural process of photosynthesis. This led us to wonder: Could there be a way to overcome excitation annihilation? The quest for a solution to this quandary inspired our work.”
Quantum interference
Quantum interference comes into play during the interaction of excitons because their wavefunctions can interact in a manner that causes either constructive or destructive interference.
It’s “often believed to be fragile,” says Roel Tempelaar, an assistant professor of chemistry at Northwestern University. “This is an exciting new direction for the use of quantum interference enabled by detailed chemical control of molecular crystals.”
Phase relationships of exciton wavefunctions—how the peaks and troughs of their wave-like properties align in space over different molecules—determine the annihilation probability.
The team discovered the annihilation process of excitons is sensitively dependent on these spatial quantum phase relationships (see Fig. 2). “The interaction between the molecules, a.k.a. dipole-dipole coupling, can yield annihilation pathways that interfere destructively (in H-aggregates) or constructively (in J-aggregates),” Huang says. “To put it simply: the ‘sign’ of the coupling—either positive or negative—dictates whether the interference will be constructive or destructive, and this can significantly influence the fate of excitons when they encounter each other. In H aggregates, they simply pass by each other without annihilation.”
As far as the key takeaway of the team’s work, Huang says it’s “the profound impact of quantum phase on the behavior of excitons, and the potential for this understanding to influence the design of molecular materials from the bottom up.”
They discovered that quantum phase relationships of excitons can be manipulated by subtly altering the structure of molecules—by adding different side-groups to perylene diimide (an industrial dye) molecules. These modifications cause the molecules to crystallize in different structures, which dramatically changes the quantum phase relationships of the excitons.
“One of the most exciting aspects of this work is the striking alignment between our experimental observations and theoretical predictions,” says Huang. “There’s always a degree of uncertainty when venturing into new territory, as we were with this project. It’s exhilarating to see the theoretical predictions of exciton annihilation so closely match the behaviors we were observing in the lab.”
There were several ‘a-ha’ moments during the project, but the one that stands out to Huang most is when they first observed the dramatic difference in exciton annihilation rates in different structures. “Seeing the experimental data so clearly demonstrate this effect was both surprising and incredibly satisfying,” she says.
Time-resolved photoluminescence microscopy
One significant challenge the team faced was to separate the impact of exciton diffusion from exciton annihilation, since the conventional view had been to assume annihilation as an incoherent, diffusion-limited process.
“Understanding this distinction was crucial to our work because we aimed to explore the role of quantum phase relationships in exciton annihilation, independent of their diffusion,” says Huang.
To address this challenge, they turned to time-resolved photoluminescence microscopy, which allowed them to observe the dynamic behavior of excitons in molecular materials with high temporal and spatial resolution.
“This way, we could measure both the diffusion constants that describe the motion of excitons and the annihilation rates that represent their interactions,” Huang says.
Harnessing quantum interference
By harnessing quantum interference, “we can achieve a condition where low annihilation coexists with high exciton concentrations and mobilities,” says Huang. “This is a major stride forward, because traditionally, high exciton densities were believed to inevitably lead to high rates of annihilation, which can negatively impact the efficiency of energy and information transport.”
The potential applications of the team’s work are enormous. In photovoltaics, for example, reducing the exciton annihilation while maintaining high exciton densities could significantly improve the efficiency of solar cells. Similarly, in the field of light-emitting diodes, controlling exciton behavior could lead to brighter and more energy-efficient devices.
Furthermore, the work could be beneficial in the development of quantum communications. Excitons, in essence, act as information carriers within these systems. The ability to control their densities and annihilation rates might pave the way for the design of more robust and efficient quantum systems.
Next, Huang and colleagues will focus on making quantum interference effects more robust against fluctuations and disorder, which present a challenge to the practical application of their findings.
“Quantum interference effects are often highly sensitive to factors such as temperature, vibrations, and imperfections within the material structures,” Huang says. “These disturbances can disrupt the quantum phase relationships among excitons and compromise the structure or destructive interference patterns we aim to achieve.”
They’ll tackle this challenge by exploring how to design molecules to better withstand these disturbances. “This involves delving deeper into the molecular structures and the relationships between their physical properties and the resulting quantum interference effects,” Huang says.
FURTHER READING
S. Kumar et al., Nat. Chem. (2023); https://doi.org/10.1038/s41557-023-01233-x.
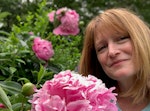
Sally Cole Johnson | Editor in Chief
Sally Cole Johnson, Laser Focus World’s editor in chief, is a science and technology journalist who specializes in physics and semiconductors. She wrote for the American Institute of Physics for more than 15 years, complexity for the Santa Fe Institute, and theoretical physics and neuroscience for the Kavli Foundation.