New tool determines characteristics of light-based quantum states
A group of physicists at the Paderborn University’s Institute of Photonic Quantum Systems in Germany came up with a relatively simple way to determine the characteristics of light-based quantum states. To pull off this feat, they use single-photon detectors—devices to detect individual light particles—for homodyne detection, a method frequently used in quantum optics to explore the wave-like nature of optical quantum states.
The method was developed by the Mesoscopic Quantum Optics working group, led by Professor Tim Bartley, and they showed its ability to characterize optical quantum states—which make it an essential tool for quantum information processing.
“On a basic physics level, my group likes working with photons because, as fundamental energy quanta, we can use them to study quantum mechanics in a very experimentally accessible way,” says Bartley. “Developing the correct measurement methods to uncover quantum mechanical phenomena with photons is an important aspect of this process.”
Developing tools to measure these properties is becoming increasingly relevant and, by building upon their results, the aim is to exploit the quantum mechanical properties of photonic quantum states to perform tasks more efficiently.
“We wanted to develop a technique to measure the quantum mechanical properties of electromagnetic fields at the single-photon level,” says Bartley. “Homodyne detection is a well-established method to do this, but conventional homodyne detectors are typically based on semiconductor detectors (photodiodes).”
Bartley’s group decided to explore whether superconducting nanowire single-photon detectors (SNSPDs) can also be useful for this task.
“This is interesting since these detectors have an extremely high signal-to-noise ratio, which is important in homodyne detection,” Bartley says. “But it’s not obvious that they’re suitable, since they’re mainly operated in the ‘click/no click’ regime—they don’t respond linearly to incident light, but rather detect the presence or absence of photons. Nevertheless, we could show that by using the count rate of these devices operated in the low-intensity continuous-wave regime, they can recover the quantum noise properties of a light field.”
Simple interferometer setup
The group’s setup is relatively straightforward: a simple unbalanced interferometer, with one arm defined as a probe state, and the other as a phase reference (a weak coherent state).
“We look at the difference in count rate of the two output ports as measured by our superconducting detectors, and the noise of this difference signal is proportional to the fundamental quantum noise of the probe state,” Bartley explains. “The laser we use is a continuous-wave diode laser, and our challenges were mainly analyzing the data and moving from single-photon click rates to electric field quadratures.”
At a high level, the main benefit of photonic detector engineering at the quantum level is to work at the limits of optical measurement. It involves learning the most about a system using only a few resources—measurement time, light intensity, etc.
“Since photons are the fundamental quanta of optical energy, measurements at this level are already ‘quantum limited.’ We want to push the boundaries of the properties of optical fields that can be measured at the single quantum level and develop tools that are also sensitive to nonclassical physics within this regime,” Bartley says. “This, in turn, may be exploited for new applications.”
It was very satisfying for the group to see the results come through from the analysis “showing that we are indeed quantum noise limited, and that this noise clearance above shot noise is so high,” says Bartley. “This was confirmation of the ‘a-ha’ idea that count rates can be used to run these detectors within the linear regime. My Ph.D. students Maximillian Protte and Timon Schapeler worked very hard to get the experiment running, so to see these results was a nice reward for their hard work.”
Despite the relative simplicity of their setup, “there were several ‘moving parts’ that needed to work in concert to produce good data,” Bartley adds. “When measuring noise, one of the biggest challenges is to identify all imperfections and understand their effect on the results. This also feeds into the analysis, and we were helped significantly by Professor Jan Sperling, also at Paderborn University, who translated the click statistics from the measurements to the field quadrature picture required to interpret the fundamental quantum noise. It was a real team effort!”
Another tool for the quantum optical toolbox
Homodyne detection is already a widely used technique for communications systems and fundamental measurements, such as gravitational wave detection.
“While our approach is somewhat niche, we’ve shown that such measurements can be made with a very low-intensity local oscillator, which may be relevant in applications where available optical power is limited,” Bartley says. “Looking further into the future, this technique is another addition to the quantum optical toolbox, particularly when working with continuous variables of quantum states, which has applications in quantum computing and communications.”
In other recent work, Bartley’s group showed how photon-number information can be extracted directly from commercial SNSPDs.
“This technique can be directly applied to the homodyne detection experiment, although we need to reconcile the operating conditions of each technique—pulsed vs. continuous-wave,” Bartley says. “Nevertheless, doing so will allow us to perform high-noise-clearance, low-light-level homodyne on a wider variety of quantum states, such as squeezed states, which are the building block of all quantum computation and sensing applications with continuous variables.”
FURTHER READING
M. Protte, T. Schapeler, J. Sperling, and T. J. Bartley, Optica Quantum, 2, 1–6 (2024); https://doi.org/10.1364/opticaq.502201.
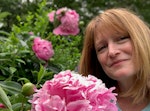
Sally Cole Johnson | Editor in Chief
Sally Cole Johnson, Laser Focus World’s editor in chief, is a science and technology journalist who specializes in physics and semiconductors. She wrote for the American Institute of Physics for more than 15 years, complexity for the Santa Fe Institute, and theoretical physics and neuroscience for the Kavli Foundation.