Neuroscience/Near-Infrared: A new optical window opens for deep brain imaging
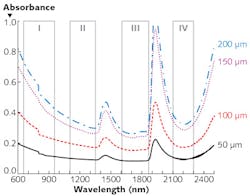
Optical imaging is critical to life sciences because it enables superior spatial resolution. But because scattering and absorption are inherent barriers to light propagation in tissue (scattering blurs images while absorption reduces availability of photons), a key goal is maximizing optical imaging depth. This is true for bioimaging in general, and neuroimaging in particular.
Because the scattering coefficient decreases with increasing wavelength, light of longer wavelengths is able to penetrate deeper into tissue. Compared with wavelengths in the visible range, near-infrared (NIR) light at 650–950 nm—commonly known as the "therapeutic window," and what we call Optical Window 1—has been recognized as advantageous for its reduced absorption and scattering. The availability of low band gap silicon-based photodetectors has enabled scientists in recent years to leverage these advantages for deep-tissue imaging. But while technology has limited access to wavelengths beyond 950 nm, new advances—specifically suitable detectors and femtosecond laser sources—offer greater potential. Such tools have facilitated research, revealing three additional NIR optical windows and corresponding facilitation of deeper imaging. New work shows the promise of one window in particular for optimal imaging of brain tissue.1 The work examined the transmission of light through brain tissue, and applied theoretical study to estimate optical parameters and determine the key wavelengths and attenuation coefficients in four NIR ranges.
Using the tools
When propagating through tissue, light separates into ballistic, snake, and diffusive components.2,3 Multiphoton microscopy enables deep-tissue imaging by using the ballistic component of NIR light to excite fluorescence in Optical Window 1. Exciting fluorescent agents to their second singlet (S2) state, where both the excitation and emission wavelengths fall within the optical window, enables deeper imaging.4 The difficulty of the S2 pumping technique lies in a dearth of appropriate contrast agents. An alternate solution is to apply longer NIR wavelengths for tissue imaging, so that—in line with Rayleigh and Mie theories—scattering and absorption are further decreased.
Researchers at City University New York reported imaging of tissues in three longer-wavelength NIR regions: Optical Window II (1100–1350 nm), Window III (1600–1870 nm), and Window IV (centered at 2200 nm).5 Even more recent work investigated key properties of light in the three windows compared with the traditional therapeutic window. It calculated corresponding attenuation length (lt) using rat brain sliced at four different thicknesses (50, 100, 150, and 200 μm). Using a UV/VIS/NIR spectrophotometer that measured light transmission through each slice, we were able to identify the optical window with the lowest scattering and noise, and optimized absorption—that is, the window best suited for imaging brain tissue.
Transmittance and absorbance
A study of peak transmittance for the 50, 100, 150, and 200 μm slices in the various wavelength ranges (summarized in the table) demonstrates that all three of the "new" optical windows enable deeper imaging than the standard therapeutic window of 650–950 nm. And it shows that Window III (1600–1870 nm) is best at facilitating peak transmittance. Window III turns out to be best in terms of absorption as well: Looking at measured absorbance of light through the four tissue thicknesses in the various windows reveals a trough of absorbance spectra in that range (see figure). For these reasons, we call Window III the Golden Window for brain imaging.
The ballistic photons in scattering media are governed by the Beer-Lambert law, which can be adjusted to incorporate diffusive mode. We used this equation to evaluate the role that each mode plays in light transmission through brain tissue: We performed simulations in the four optical windows and compared the results with our peak transmittance measurements. The model simulation results matched well with our experimental results across the board. The results also suggested that ballistic mode played a dominant role in our experiment, and that the role diffusive mode played is minimal.
Other studies examining Window III have confirmed its value. The work at City College New York comparing all four NIR optical windows also found Window III to be optimal for imaging.5 Scientists at Purdue University (West Lafayette, IN) demonstrated that Window III allows deeper tissue penetration in their study of overtones and combination modes in the methyl groups of proteins, amino acids, and lipids.6 And work by InfraReDx Inc. (Burlington, MA) suggests that Window III is optimal for exploring the cholesterol and collagen content of different types of tissue.7
Brain tissue is distinct from other tissue types. Compared with muscle, for instance, it contains twice as much lipid, but less than half as much protein.8 More work is needed to determine the impact of these and other tissue components on optimal wavelength, and to compare neural and other tissues. This work promises to facilitate imaging of brain tissue in space. New CMOS cameras and photodetectors based on InSb will also help to improve imaging of blood vessels within the brain, and extracellular space, in vivo. We look forward to further work advanced by these tools.
REFERENCES
1. L. Shi, L. A. Sordillo, A. Rodríguez-Contreras, and R. Alfano, J. Biophoton., 9, 1–2, 38–43 (2016).
2. L. Wang, P. P. Ho, C. Liu, G. Zhang, and R. R. Alfano, Science, 253, 769–771 (1991).
3. R. R. Alfano, W. B. Wang, L. Wang, and S. K. Gayen, PHOTONICS: Scientific Foundations, Technology and Applications (ed. D. L. Andrews), John Wiley & Sons, Hoboken, NJ (2015).
4. L. Shi et al., J. Biomed. Opt., 19, 066009 (2014).
5. L. A. Sordillo, Y. Pu, S. Pratavieira, Y. Budansky, and R. R. Alfano, J. Biomed. Opt., 19, 056004 (2014).
6. P. Wang, H. W. Wang, M. Sturek, and J. X. Cheng, J. Biophoton., 5, 25–32 (2012).
7. J. D. Caplan, S. Waxman, R. W. Nesto, and J. E. Muller, J. Am. Coll. Cardiol., 47, C92–C96 (2006).
8. H. McIlwain and H. S. Bachelard, Biochemistry and the central nervous system, Churchill Livingstone, New York (1985).
Lingyan Shi
Dr. Lingyan Shi is currently an Associate Professor in the Shu Chien-Gene Lay Department of Bioengineering at UC San Diego. Her research focuses on developing high resolution optical spectroscopy and imaging platforms, and its applications for studying metabolic dynamics in aging and diseases. She discovered the “Golden Window” for deep tissue imaging and developed bioorthogonal metabolic imaging platforms that combine deuterium probing and stimulated Raman scattering (DO-SRS using heavy water and STRIDE with D-glucose) for visualizing metabolic activities in situ. The Shi group transformed SRS into a super resolution microscopy with chemical selectivity by developing Adam optimization-based Pointillism Deconvolution (A-PoD) methods. Dr. Shi holds six awarded patents. She won the Blavatnik Regional Award for Young Scientist in 2018; the Hellman Fellowship Award 2021; the “Rising Star Award” by Laser Focus World, and the “Rising Star Award” by Nature Light Science & Applications in 2021; the “Advancing Bioimaging Scialog Fellow” by RCSA and the Chan Zuckerberg Initiative in 2021, 2022, and 2023; and the Sloan Research Fellow Award in Chemistry 2023.
Dr. Shi has been mentoring graduate and undergraduate students to help them achieve excellence in academic work and become successful engineers and scientists. She plans to continue making additional contributions by enhancing more participation of underrepresented groups from the UC San Diego communities. She has been teaching core undergraduate and graduate courses in the Shu Chien-Gene Lay Department of Bioengineering at the UC San Diego Jacobs School of Engineering.