Photonics Products: Spectrometers: Imaging spectrometers examine the world around us
There are many ways to classify optical spectrometers—by their size, wavelength range, or optical configuration, or whether they work via absorption, emission, transmission, or scattering, for example. But one of the most fundamental divisions is between imaging and nonimaging spectrometers. If you're analyzing a uniform sample or looking at the spectral properties of a single light source, then nonimaging is fine. But if you need to get spectral details for every point across a scene or a medical specimen, then you need an imaging spectrometer.
Imaging spectrometers themselves come in different forms—for example, multi- or hyperspectral instruments, which often characterize externally illuminated objects such as landscapes, goods on an assembly line, or objects under a microscope; and Raman instruments, which use an internal laser to illuminate the object or area under test, then analyze the resulting Raman-scattered (and wavelength-shifted) light.
At least some of the technology inside a particular spectrometer, which includes the optics, the type of sensor array, the electronics, and the software, is at partly unique to the instrument's manufacturer; many imaging spectrometers are tweaked—or even specifically designed—for a certain application. Because such technology is often specific to an instrument's maker, this article will cover the topic of imaging spectrometry by focusing on a few instrument manufacturers one at a time.
Many configurations for science
In science, and in particular the biosciences, imaging spectroscopy finds use in microspectroscopy, multichannel plasma analysis, photoluminescence and electroluminescence, Raman microscopy and spectroscopy, time-domain-fluorescence imaging microscopy, time-resolved imaging and spectroscopy, fluorescence spectroscopy, and other applications. Horiba JobinYvon (Edison, NJ) produces a range of imaging spectrometers that are paired with the company's scientific cameras.
Horiba designs and manufactures imaging spectrometers varying in focal length from 70 to 1250 mm. "As diffraction gratings are a core technology of Horiba JobinYvon, we have the ability to optimize each design to best match the experimental requirements of wavelength range, resolution, throughput, size, and cost," notes Joanne Lowy, Horiba's marketing manager.
The necessity for a large choice of designs is driven by applications, cost, and size constraints set by the researchers, says Lowy. In an example of one design, Horiba produces Czerny-Turner imaging spectrometers with fully aberration-corrected reflective optics that the company says are free of rediffracted light (the Czerny-Turner design, the basis for many imaging-spectrometer optical layouts, has two mirrors that image an entrance slit onto an exit slit; a diffraction grating between the mirrors disperses the light). In another design, Horiba offers supercorrected concave holographic grating-based spectrometers in which the concave grating performs the function of collimation, dispersion, and focus in one element.
The shape of Horiba's iHR550 imaging spectrometer is dictated by the requirements of the spectrometer, explains Lowy. All-oversized reflective optics eliminate chromatic aberration (their large size provides high throughput); toroidally corrected optics reduce aberrations; and careful selection of the angle of incidence eliminates rediffracted light. The last is of particular importance, as systems that suffer from rediffracted light, such as criss-cross Czerny-Turner spectrometers, will not produce pure spectra at their output foci, which can lead to erroneous spectra or images.
Unlike typical imaging spectrographs, which measure over only a narrow spectral band and (due to their optical design) one optical output, the iHR550 has multiple entrance ports, multiple gratings with on-axis rotation, multiple array exit ports with wide focal planes accessible outside the instrument's casting. With selection of appropriate gratings, the instrument has a spectral range from the deep-UV (122 nm) to the far-IR (40 μm).
Uses for the iHR550 named by Lowy include multitrack plasma analysis (which requires high resolution and imaging quality); direct coupling to a microscope for photon-starved applications such as Raman spectroscopy; broadband-emission applications such as photoluminescence; and time-resolved applications such as fluorescence-lifetime imaging microscopy (FLIM).
Abolishing astigmatism
The Czerny-Turner configuration is not simply a commonly used imaging-spectrometer design; it also serves as a springboard for inventive improvements. One of these is a Czerny-Turner-based configuration developed by Princeton Instruments (Acton, MA) that contains the addition of a Schmidt corrector to its optics; this is a transmissive plate with an aspheric surface that corrects aberrations (added to a telescope, a Schmidt corrector plate is designed to eliminate spherical aberration).
The company's imaging spectrograph, the IsoPlane 320, has zero astigmatism at all wavelengths across the entire focal plane. When used in multichannel spectroscopy, in which a number of fiber-optic probes (the "channels") are placed across the image field to collect information, the astigmatism-free design allows up to 200 channels, according to Jason McClure, chief scientist at Princeton Instruments and designer of the optics. The design, which is patent-pending, also boosts sensitivity by focusing more photons onto fewer pixels.
The IsoPlane 320 is used, for example, in tip-enhanced Raman spectroscopy, studies of carbon nanotubes, plasmonic structures, and quantum dots. The ability of the IsoPlane to image dozens of optical fibers greatly enhances the spatial resolution available for imaging. In single-molecule tip-enhanced Raman spectroscopy (SMTERS), both the sample and signal are minuscule; the IsoPlane is the only spectrograph to date that has successfully been used in this technique, says Debby Flint-Baum, marketing communications manager at Princeton Instruments.
Using the IsoPlane 320 for this purpose, the Van Duyne group at Northwestern University has combined SMTERS and scanning tunneling microscopy (STM) to obtain unprecedented sensitivity and spatial resolution in obtaining Raman spectra of single rhodamine 6G molecules, advancing the understanding of excited-state dynamics in adsorbate-substrate interactions.1
Handheld hyperspectral
Hyperspectral cameras divide the light spectrum into many small wavelength bands (compared to traditional cameras, which only have three bands-red, green, and blue); spectrometers based on this technology thus can give very detailed information about the material constitution of an imaged object. Based on its own volume-phase-grating (VPG) technology, BaySpec (San Jose, CA) has developed two closely related battery-powered handheld hyperspectral imaging spectrometers that enable field-based uses in agriculture, food inspection, hazardous-materials detection, and surveillance.
Both are VPG-based, which gives them an efficiency of up to 99%, along with high stability and repeatability, as there are no moving parts. The instruments can be configured for any spectral range within 400 to 1100 nm, with a spectral resolution up to 0.01 nm. Coupled with a high-speed camera, the system can generate hyperspectral image cubes with up to 2048 spectral bands in a single scan.
BaySpec's handheld hyperspectral imager, the OCI-1000, integrates the spectral dispersing element (which has more than 100 bands covering the 600 to 1000 nm range) on an image sensor at the level of the chip itself, eliminating the need for the large, expensive optics that are used on traditional systems. The instrument weighs less than 0.5 lb. and can be installed almost anywhere from UAV drones to conveyor belts in production lines, or just used as a handheld unit, says Lin Chandler, spectroscopy product manager at BaySpec.
The similar (and, at 0.8 lb., just slightly heavier) OCI-2000 snapshot imager goes one step further, simultaneously dispersing 2D images into multiple spectral bands (currently 32 bands covering the 600 to 1000 nm range), eliminating the need for mechanical scanning. Because a single "snapshot" produces an entire multispectral image data cube, true multispectral imaging can be done at video rates.
In environmentally hazardous areas, most hazardous wastes or emissions are invisible and hard to detect remotely; with their standoff capability, these hyperspectral imagers enable in-the-field detection and identification of these chemicals. Chandler adds that one of the novel applications in development for these hyperspectral imagers is as a noninvasive diagnostic tool in medical fields. For instance, such an instrument can achieve an accurate hyperspectral representation of the anatomy of any living organism without disturbing it.
Photon conservation
By efficiently making use of photons, an imaging spectrometer not only shortens data acquisition times; it can also minimize damage to photosensitive samples. Andor (Belfast, Ireland) is known for its low-light scientific cameras; the company also produces imaging spectrometers that conserve photons.
The company's Czerny-Turner instruments have motorized multiport, multigrating platforms with toroidal optics for astigmatism correction of aberrations inherent to CT design; these spectrometers provide good multitrack spectroscopy capabilities, as well as good sample image relay at "zero order" in microspectroscopy configurations (allowing the sample to be visualized and spectral analysis to be performed through the same optical path with one detector), according to Marion Mathieu, the company's digital marketing executive.
Andor also makes instruments with a transmission-optics configuration and low-stray-light volume-phase-holographic (VPH) gratings, resulting in high collection efficiency, throughput, and multitrack-spectroscopy capabilities across a large focal plane. The company's third type of instrument is hyperspectral, based on either a "grism" (combined grating and prism) transmission configuration or an aberration-corrected reflective diffraction-grating configuration.
The instruments can be used in transmission or reflection-based absorption spectroscopy, Raman spectroscopy at wavelengths from the UV to the near-IR, micro-Raman and microfluorescence, photon counting, and single-molecule spectroscopy (all requiring efficient use of photons); also for plasma studies and for laser-induced breakdown spectroscopy (LIBS).
Applications are many, including biomedical screening and diagnosis, material analysis (polymers, metals, semiconductors, nanoparticles such as carbon nanotubes, quantum dots, and diamonds), chemical-reaction monitoring, pharmaceutical or petrochemical process control, mineralogy, analytical cell biology, security and defense (such as standoff chemical or explosive detection), and fundamental physics research.
As in any optical system, combining high light throughput (low f numbers) with high resolution (low aberrations) is challenging. Andor's VPH-based transmissive-optics imaging spectrometer, called the Holospec, is an on-axis high-throughput instrument with imaging-corrected optics that allow both high-density multi-track spectroscopy (clear separation of individual channel images from densely packed fiber-optic bundles) and high throughput, says Mathieu. The f/1.8 aperture allows 100% light collection from fiber optics with a numerical aperture (NA) of 0.22.
"Up to 32 100-μm-core fiber channels can be individually resolved over a 4-mm-high sensor with low crosstalk, despite the high-density fiber-bundle configuration," notes Mathieu.
Applications include intrinsically photon-starved experiments such as quantum-dot photoluminescence, micro-Raman of biosamples, microphotoluminescence of carbon nanostructures, plasmonics spectroscopy of light-harvesting complexes or organic light-emitting diodes (OLEDs), cathodoluminescence, and stand-off chemical detection.
The Holospec is also used when acquisition time is a constraint (gathering enough photons in short periods of time while maintaining a meaningful signal-to-noise ratio)—for example, in microspectroscopic chemical mapping, microfluidics such as spectrally resolved flow cytometry, and on-line process control. In addition, efficient photon use minimizes photodamage of photosensitive samples such as live cells or luminescent biotags, protecting them from photodegradation and phototoxicity.
Imaging spectrometers see near and far
While spectrometers do perfectly well sitting in labs and eyeing microscopic samples, they can also see the world—for example, from the vantage point of an unmanned aerial vehicle (UAV); Headwall Photonics (Fitchburg, MA) makes instruments that do either. The company produces both hyperspectral and Raman imaging spectrometers, with the hyperspectral version the one that gets to take the airborne route (see Fig. 1).
"The type of hyperspectral technology we deploy is commonly known as 'pushbroom,' meaning that motion needs to occur," says David Bannon, Headwall's CEO. "Either the sensor moves about the field of view (as it would in an aircraft), or the scene moves beneath a stationary sensor (as it would in an in-line inspection deployment)."
Headwall's hyperspectral instruments have an athermalized design, making them fully functional in demanding environments such as those that UAVs endure, and have almost no moving parts—again, for reliable performance while aloft. The instruments have aberration-corrected imaging to provide high spatial and spectral resolution across a wide field of view. "They have excellent image fidelity off to the sides," says Bannon.
Headwall makes its own diffraction gratings that provide this aberration-corrected imaging; this aids in designing a package that tightly integrates optics, electro-optics, electronics, and software, but also aids in customization; the company also customizes its software when needed, in some cases for the specific application (for example, UAV platforms) and in others for a specific customer (for example, to identify foreign objects in food processing).
There are four key areas served by Headwall's imaging spectrometers: remote sensing, most notably UAV and other airborne applications, but also including satellite payloads that offer spectral imaging as an alternative to the standard radar data set; military and defense, both airborne and handheld; medical and biotech (establishing noninvasive diagnostic techniques as well as laboratory applications for microscopy); and industrial inspection. As for the last, "Imaging spectroscopy is the next generation of machine-vision capability," says Bannon.
Some specific uses include inspection of poultry, where hyperspectral imaging spectrometry can actually spot diseased versus healthy poultry; document and artifact verification and analysis using nondestructive illumination, which allows curators and other experts to learn more about their treasures, and noninvasive medical analysis and interpretation—for example, identifying and differentiating between types of skin cancer.
However, UAVs are what's making the news these days. "Headwall's sensor design is geared toward airborne deployment," says Bannon. "Users of hyperspectral sensors aren't always white-coated scientists. They need help optimizing these elements so that they work well together: 1) the sensor; 2) the hyperspectral data processing unit that collects the incoming data and manages it, also 'instructing' the sensor when and where to operate; the software (Hyperspec III) needs to not only manage the data and sensor operation, but do so with an easy-to-assimilate GUI; 3) GPS and inertial-navigation system (INS); and 4) cabling/cooling/overall integration."
As a further step into the UAV arena, Headwall has begun selling a package consisting not just of its Micro-Hyperspec sensor in the airborne configuration—but also the UAV that flies the sensor system.
Instruments from EUV to LWIR
Imaging spectrometers can be designed for use in just about any region within what's commonly called the optical spectrum, including extreme ultraviolet (EUV), deep ultraviolet (DUV), UV, visible (Vis), near-IR (NIR), or short-wave, mid-wave, and long-wave IR (SWIR, MWIR, and LWIR). McPherson (Chelmsford, MA) makes them all.
McPherson's instruments collect spectra from multiple, spatially distinct channels. They can be one of several different optical designs; each is optimized for a particular spectral range or particular type of application, says Erik Schoeffel, marketing and sales at McPherson.
For example, MWIR and LWIR 2D spectroscopy applications favor low-dispersion prism-based systems. These disperse many microns of spectral range on an array detector and have high throughput without multiple-order effects, albeit relatively low spectral resolution, explains Schoeffel.
In contrast, UV-Vis-NIR spectroscopy applications gravitate towards diffraction-grating-based, high-resolution, long-focal-length instruments. These tend to use fiber optics. These disperse a couple of nanometers spectral range on the array detector and have high line symmetry and spectral resolution. The DUV range (above 105 nm to IR) uses essentially the same UV-Vis technology but in a vacuum environment. "This obviously excludes wavelength-range-limiting fiber optics and requires a line of sight or optics to first image the source or experiment at the entrance aperture," says Schoeffel.
Finally, EUV (above 8 nm or so) uses a dramatically different instrument layout, since all optics function at grazing incidence angles. The outcome, however, is very similar to UV-Vis instruments in that the array can spatially distinguish parts of the source or experiment and collect spectra simultaneously. Line of sight is required for the shortest wavelengths.
"Probably the most common imaging spectrometer application we encounter is high-temperature plasma diagnostics," says Schoeffel. "These applications most often use fiber-coupled UV-Vis-NIR instrument designs and less frequently the DUV and EUV designs. High-resolution spectroscopy measurements of emission-line Stokes shift or Doppler broadening allow noninvasive measurement of hot plasmas. The experiments tend to be large enough to allow numerous fiber-optic probes to be deployed and monitor many different points in a reaction or features: impurities, ionization, temperature, and the formation and confinement properties."Typical systems for high-temperature plasma diagnostics are built on 0.67, 1, or 1.3 m focal-length instruments. They provide an optical bench suitable for spectral measurements to an approximately 0.02 nm FWHM linewidth, or wavelength shift measurements. They are integrated with fast, sensitive array detectors and multiple fiber inputs. Multiplexing fiber inputs allow a single instrument to monitor high-resolution emission line profile or shifts at up to 100 plasma points simultaneously (see Fig. 2).
Quantitative color measurement
One property of light in the visible region that can be extracted from imaging spectrometry data is color—important in fields such as printing, textiles, and artwork. Tricor Systems (Elgin, IL) makes an imaging spectrophotometer, the Model 600, especially to analyze color (see Fig. 3).In typical use, the Model 600 acquires 20 images in 20 nm wavelength bands from 480 to 780 nm, explains Tom Allen, vice president of sales and marketing at Tricor. "The resulting 1280 × 960 pixel image contains 1.2 million sets of spectral curves from 480 through 780 nm, one for each pixel," he says. "The most useful tool, to date, is the full image comparison. The software allows the user to compare full images pixel by pixel in order to quantify the differences between the images. This is valuable to industries that need reproducibility to match a standard."
The images can also be compared by means of a so-called virtual spot spectrophotometer (VSS), which allows the user to define the measurement spot size, freeing the user from hardware spot-size limitations. In one implementation, the user manually draws a rectangular aperture on the computer screen over the image to extract a single convolved (or averaged) color.
A second implementation includes the ability to perform a full-frame "delta-E" (pixel-by-pixel quantitative color difference between two images) analysis using a user-defined aperture size. The resulting delta-E map allows the user to visualize the entire surface of, for example, a print coming off a production line, and how it compares in its entirety to a master print.
Artwork such as lithographs, oil paintings, photographs, and textiles is generally nonuniform in nature, which, when color measurements need to be done, calls for the ability to average over variable-sized areas. When measuring artwork, variables such as temperature and humidity conditions can (if only occasionally) influence dyes and therefore should be consistent when measuring; light sources should be controlled (especially their UV output) to prevent damage during measurement.
Analysis can take many forms. Discrete color measurements can be done over large (or small) areas by selecting a region of interest. In just one example, a mapping could consist of four colors, with each color representing a specific range of delta-E values. The resulting quantitative color-measurement maps allow a curator to compare art reproductions to stored originals, or compare an art piece to previous measurements to see if it is degrading over time.
REFERENCE
1. M. D. Sonntag et al., J. Am. Chem. Soc., 135, 17187 (2013); doi:10.1021/ja408758j.
For comprehensive listings of component vendors by product category, visit the Laser Focus World Buyer's Guide. To locate spectrometer vendors, visit the Optical Test & Measurement Equipment section.
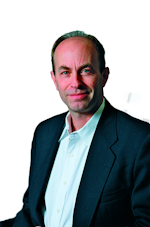
John Wallace | Senior Technical Editor (1998-2022)
John Wallace was with Laser Focus World for nearly 25 years, retiring in late June 2022. He obtained a bachelor's degree in mechanical engineering and physics at Rutgers University and a master's in optical engineering at the University of Rochester. Before becoming an editor, John worked as an engineer at RCA, Exxon, Eastman Kodak, and GCA Corporation.